Advanced Techniques for Solving Fluid Machinery Challenges
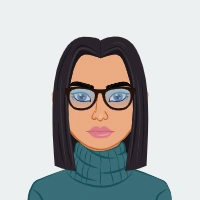
When tackling assignments related to mechanical engineering fluid machinery, students frequently encounter a diverse array of intricate and multifaceted problems involving pumps, compressors, turbines, and other related systems. These assignments require a nuanced understanding of fluid dynamics, machinery performance, and engineering standards, integrating both theoretical concepts and practical applications. Students must delve into the complex interactions between fluid flow and mechanical components, analyzing how various factors such as pressure, temperature, and material properties influence system behavior.
Addressing these complex assignments demands a thorough approach that encompasses several critical steps. First, students must engage in detailed research, utilizing scholarly articles, industry reports, and technical standards to build a solid foundation of knowledge. This includes understanding the fundamental principles governing fluid machinery, such as the behavior of fluids under different conditions and the impact of these conditions on machinery performance.
Additionally, students need to apply relevant engineering standards and guidelines to ensure their analyses are grounded in best practices. This involves examining how established norms and regulations affect the design and operation of fluid machinery. For instance, understanding API standards for pump design or ASME guidelines for turbine performance can provide valuable context for solving specific problems.
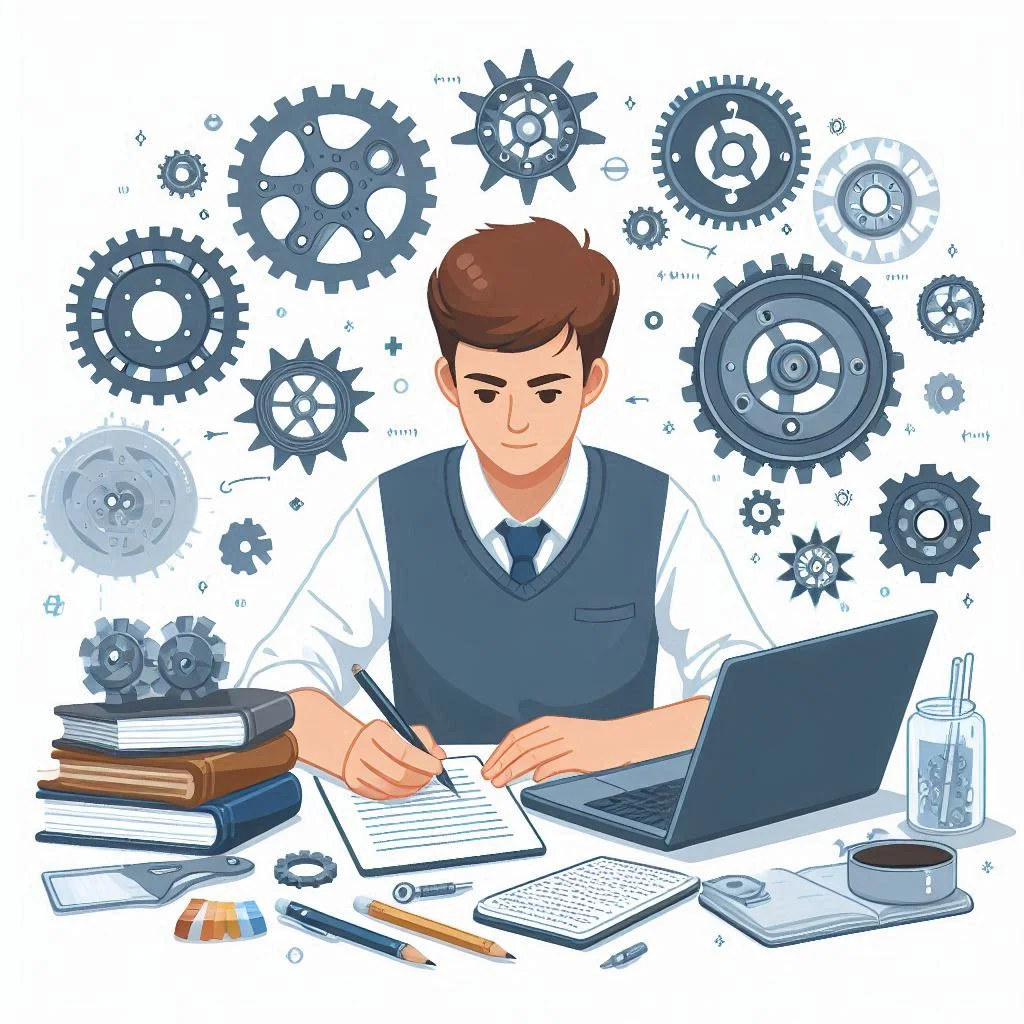
Moreover, a systematic approach is essential for effectively tackling each component of an assignment. This means breaking down complex questions into manageable parts, addressing each element with a detailed and methodical analysis. For example, when exploring cavitation in turbomachinery, students must investigate the underlying causes, evaluate potential mitigation strategies, and assess the effects on performance and reliability.
In addition to technical analysis, students should consider the broader implications of their findings, such as the potential impact on system efficiency, safety, and operational costs. This holistic perspective ensures that assignments are not only technically accurate but also relevant to real-world applications.
"By combining rigorous research, adherence to engineering standards, and a comprehensive analytical approach, students can effectively tackle complex assignments in mechanical engineering fluid machinery. This structured methodology not only enhances their problem-solving skills but also prepares them for future challenges in the field, making fluid mechanics assignment help service an invaluable resource for their academic success."
Understanding Cavitation in Turbomachinery
Cavitation is a critical phenomenon in the field of turbomachinery, particularly when dealing with high-performance systems such as turbopumps used in aerospace and industrial applications. This complex process occurs when the pressure of a fluid drops below its vapor pressure, leading to the formation of vapor bubbles. As these bubbles travel through the fluid stream, they can collapse violently when they encounter regions of higher pressure, resulting in shock waves that can cause significant damage to the turbomachinery components.
"Understanding cavitation is essential for engineers and designers to prevent performance issues and ensure the longevity and reliability of machinery. For those seeking detailed explanations and assistance with related topics, taking help from experts can provide valuable insights and solutions. Addressing cavitation effectively involves optimizing system design and operating conditions to avoid the conditions that lead to bubble formation and collapse. If you're looking to solve your mechanical engineering assignment, understanding cavitation and how to manage it is a crucial skill."
Introduction to Cavitation
Cavitation is a dynamic process that affects various types of fluid machinery, including pumps, compressors, and turbines. The phenomenon is particularly concerning in turbomachinery, where it can lead to severe operational problems. When fluid pressure decreases to a point where it reaches the vapor pressure, bubbles or cavities form within the liquid. These cavities can rapidly collapse as the fluid pressure increases downstream, producing high-intensity shock waves. The resulting mechanical forces can cause pitting, erosion, and vibration, which degrade the performance and structural integrity of the machinery.
Causes of Cavitation in Turbomachinery
Cavitation in turbomachinery can be triggered by several factors:
- Low Inlet Pressure: When the pressure at the inlet of the pump or turbine falls below the vapor pressure of the fluid, cavitation is likely to occur.
- High Fluid Velocity: Increased fluid velocity can lower the pressure in certain areas, creating conditions conducive to cavitation.
- Improper Design: Inadequate design of the turbomachinery, such as improper blade angles or insufficient suction head, can exacerbate cavitation risks.
Implications of Cavitation
The impact of cavitation on turbomachinery is multifaceted:
- Performance Degradation: Cavitation can lead to a reduction in pump efficiency and capacity, as the collapsing bubbles disrupt the smooth flow of the fluid.
- Mechanical Damage: The shock waves generated by cavitation can cause significant damage to components, leading to erosion of surfaces, reduced lifespan, and increased maintenance costs.
- Noise and Vibration: Cavitation often results in increased noise and vibration, which can affect the overall stability and operation of the machinery.
Mitigation Strategies
To address cavitation, engineers employ various strategies:
- Design Improvements: Enhancing the design of turbomachinery to ensure adequate pressure margins and avoid conditions that lead to cavitation.
- Operational Adjustments: Modifying operating conditions, such as reducing fluid velocity or increasing suction pressure, to minimize the risk of cavitation.
- Material Selection: Using cavitation-resistant materials and coatings to reduce the impact of cavitation-induced erosion.
Understanding and mitigating cavitation is crucial for maintaining the performance and reliability of turbomachinery. By addressing the underlying causes and implementing effective design and operational strategies, engineers can prevent cavitation-related issues and ensure the efficient operation of their systems. This knowledge is vital for anyone involved in the design, operation, and maintenance of turbomachinery, as it directly impacts the effectiveness and longevity of the equipment.
Analyzing Surge and Choke in Compressors
Surge and choke are critical phenomena in the operation of compressors that significantly influence their performance and efficiency. These phenomena are particularly relevant in the context of centrifugal and axial compressors, which are widely used in various industrial applications, including power generation, aerospace, and manufacturing. Understanding surge and choke is essential for optimizing compressor performance, ensuring system stability, and preventing potential damage. This analysis explores these concepts in detail, offering insights into their causes, effects, and mitigation strategies.
Introduction to Surge and Choke
In compressor systems, surge and choke are performance issues that occur when the compressor operates outside its designed operating range. These phenomena can lead to operational instability, reduced efficiency, and potential damage to the compressor components. Surge is characterized by a rapid and often violent oscillation in flow and pressure, while choke refers to the condition where the compressor is unable to handle the increased flow, leading to a significant drop in performance.
Surge in Compressors
Surge is a disruptive phenomenon that occurs in both centrifugal and axial compressors. It happens when the compressor experiences a flow reversal due to a significant imbalance between the pressure rise and the flow rate. Key aspects of surge include:
- Location on Compressor Map: Surge typically occurs near the low-flow limit of the compressor map, where the pressure ratio is high, and the flow rate is low. On the compressor operating map, it is represented by the boundary of the surge line.
- Effects on Performance: During a surge event, the compressor experiences a cyclic fluctuation in flow and pressure, which can lead to severe performance degradation, increased noise, and mechanical stress. The repeated pressure fluctuations can cause damage to the compressor components and reduce the overall lifespan of the equipment.
- Key Parameters: Parameters influencing surge include the pressure ratio, flow rate, and the stability margin of the compressor. Surge margin is a critical parameter that indicates the distance between the operating point and the surge line.
- Contributing Factors: Surge can be triggered by sudden changes in load, incorrect operational settings, or disturbances in the system. Factors such as inlet or outlet restrictions and system dynamics also play a role.
- Control Measures: Surge can be controlled using various techniques, such as surge control valves, variable geometry, and active control systems that adjust the compressor's operating conditions to avoid surge.
Choke in Compressors
Choke occurs when the compressor reaches its maximum flow capacity, and the flow cannot be increased further despite increasing the inlet pressure. This condition results in a drop in compressor performance and efficiency. Key aspects of choke include:
- Location on Compressor Map: Choke typically occurs at the high-flow limit of the compressor map, where the flow rate is maximized, and the pressure rise becomes constrained. On the compressor map, it is represented by the choke line.
- Effects on Performance: When a compressor is choked, it cannot handle any additional flow, leading to a significant drop in pressure ratio and efficiency. This condition can cause reduced performance and potential damage to the compressor if not managed properly.
- Key Parameters: Key parameters influencing choke include the maximum flow capacity of the compressor, pressure ratio, and the design constraints of the compressor components.
- Contributing Factors: Choke can be caused by excessive flow rates, operational conditions exceeding the design limits, or mechanical restrictions within the compressor.
- Control Measures: To manage choke, engineers may implement measures such as flow control valves, operational adjustments, and redesigning compressor stages to handle higher flow rates.
Analyzing and managing surge and choke are essential for maintaining the optimal performance and reliability of compressors. By understanding these phenomena and implementing appropriate control measures, engineers can ensure stable operation, prevent damage, and optimize the efficiency of compressor systems. This knowledge is crucial for designing and operating compressors across various applications, contributing to their overall effectiveness and longevity.
Feasibility Study for Offshore Pumping Systems
A feasibility study for offshore pumping systems is a critical process for evaluating the practicality, efficiency, and economic viability of implementing such systems in an offshore oil and gas production setting. This type of study assesses various factors including design requirements, equipment selection, and operational considerations to ensure the successful deployment of pumping systems in a challenging offshore environment. The goal is to provide a comprehensive analysis that supports decision-making and optimizes the overall performance of the offshore facility.
Introduction to Offshore Pumping Systems
Offshore pumping systems are essential for transporting production fluids from oil fields to processing facilities or storage terminals. These systems must handle a variety of challenging conditions including high pressures, corrosive environments, and long-distance transportation. A feasibility study involves evaluating the technical and economic aspects of these systems to ensure they meet operational requirements and industry standards.
Key Components of a Feasibility Study
1. Design Requirements
To determine the design requirements for offshore pumping systems, consider the following factors:
- Pressure and Temperature Conditions: Assess the maximum and minimum pressures and temperatures that the pumps must handle. For example, a separator pressure of 19 barg and a temperature of 60°C are typical considerations.
- Flow Rate: Determine the required flow rate, such as 90,000 barrels per day (bbls/day), which influences the selection and sizing of pumps.
- Fluid Characteristics: Analyze the properties of the production fluid, including viscosity, density, and the presence of solids or contaminants. These characteristics affect pump selection and performance.
2. Equipment Selection
Choosing the right pumping system involves evaluating various manufacturers and their products:
- Manufacturer Options: Identify and compare different manufacturers' pumping systems suitable for the offshore application. For instance, consider systems from established manufacturers known for reliability and performance in offshore environments.
- System Details: Detail the specifications of the selected pumping systems, including pump types (e.g., centrifugal or positive displacement), accessories (e.g., control valves, sensors), and drivers (e.g., electric motors or diesel engines).
- Cost and Reliability: Assess the cost of the pumping systems, including initial purchase, installation, and maintenance. Evaluate the reliability of the systems based on historical performance data and manufacturer warranties.
3. Operational Considerations
Address the operational challenges associated with offshore pumping systems:
- Maintenance and Accessibility: Evaluate the ease of maintenance and accessibility of the pumping systems on an unmanned offshore platform. Consider the frequency of maintenance and the availability of spare parts.
- Safety and Compliance: Ensure that the pumping systems comply with safety regulations and industry standards. Consider factors such as explosion-proof certifications, corrosion resistance, and emergency shutdown procedures.
4. Economic Evaluation
Perform an economic analysis to determine the cost-effectiveness of the offshore pumping systems:
- Cost-Benefit Analysis: Compare the costs of different pumping systems and their benefits in terms of performance, reliability, and operational efficiency. Consider both capital expenditures (CapEx) and operational expenditures (OpEx).
- Return on Investment (ROI): Calculate the potential ROI by evaluating the expected increase in production efficiency and the reduction in operational costs.
5. Feasibility Assessment
Integrate the technical, operational, and economic analyses to form a comprehensive feasibility assessment:
- Technical Feasibility: Determine if the proposed pumping systems can meet the technical requirements and perform reliably under the given conditions.
- Economic Feasibility: Assess whether the investment in the pumping systems is justified by the expected benefits and cost savings.
A feasibility study for offshore pumping systems is a crucial step in ensuring the successful implementation of these systems in challenging offshore environments. By thoroughly analyzing design requirements, equipment options, operational considerations, and economic factors, engineers and decision-makers can make informed choices that optimize performance, safety, and cost-efficiency. This comprehensive approach not only supports the successful deployment of offshore pumping systems but also contributes to the overall efficiency and effectiveness of offshore oil and gas production operations.
Tidal Turbines Technology
Tidal turbines represent an emerging technology harnessing the kinetic energy of tidal currents to generate renewable electricity. As a form of marine renewable energy, tidal turbines offer a reliable and predictable energy source due to the consistent nature of tidal movements. This technology has gained significant attention as a sustainable alternative to fossil fuels and as a complement to other renewable energy sources. Understanding tidal turbines involves exploring their operational principles, various technologies, market positioning, and future prospects.
Introduction to Tidal Turbines
Tidal turbines are underwater devices designed to capture the kinetic energy of tidal flows and convert it into electrical power. These turbines operate much like underwater wind turbines, with blades that rotate as water flows over them. The energy captured by the turbines is then transmitted to the shore through subsea cables, where it can be integrated into the electricity grid.
Types of Tidal Turbines
1. Horizontal Axis Tidal Turbines (HATTs)
- Design: Similar in concept to horizontal axis wind turbines, HATTs have blades that rotate around a horizontal axis. These turbines are typically mounted on a fixed foundation or floating platform.
- Advantages: High efficiency and established technology. Suitable for high flow tidal streams.
- Examples: The Atlantis Resources Ltd. AR1500 and the Andritz Hydro Hammerfest turbine.
2. Vertical Axis Tidal Turbines (VATTs)
- Design: VATTs feature blades that rotate around a vertical axis. This design allows the turbine to capture tidal energy from all directions without the need for precise alignment.
- Advantages: Can be deployed in varying flow directions and conditions. Typically simpler to install and maintain.
- Examples: The Tidal Generation Ltd.'s TGL vertical axis turbine.
3. Oscillating Hydrofoils
- Design: These devices use oscillating hydrofoils that move up and down with the tidal flow to generate power.
- Advantages: Potentially lower cost and less mechanical complexity compared to traditional turbines.
- Examples: The Ocean Power Technologies' PowerBuoy.
4. Crossflow Turbines
- Design: Crossflow turbines have blades that intersect the flow of the water perpendicularly, allowing them to capture energy from both the incoming and outgoing tides.
- Advantages: Efficient in areas with bidirectional tidal flows.
- Examples: The Nereus turbine designed by the company, Nereus Technologies.
Technologies and Innovations
1. Subsea Foundations
- Fixed Foundations: Includes gravity bases, monopiles, and tripod structures designed to anchor turbines to the seabed.
- Floating Foundations: Utilizes floating platforms tethered to the seabed, suitable for deeper waters where fixed foundations are impractical.
2. Control Systems
- Active Pitch Control: Adjusts the angle of turbine blades to optimize performance based on changing water speeds.
- Yaw Control: Enables the alignment of turbines to maximize energy capture by orienting them according to tidal flow direction.
3. Transmission Systems
- Direct Drive Systems: Connects the turbine directly to a generator, reducing mechanical losses and maintenance needs.
- Gearbox Systems: Uses gears to convert rotational speed to a level suitable for electricity generation, offering flexibility in design.
Market Analysis and Comparative Assessment
1. Levelized Cost of Energy (LCOE)
- Definition: LCOE represents the average cost to produce electricity from tidal turbines over their lifetime. It is a key metric for evaluating the economic viability of tidal energy projects.
- Comparison: Tidal energy generally has a higher LCOE compared to established renewable sources like wind and solar, due to the high costs of installation and maintenance. However, it is competitive with offshore wind energy, particularly in regions with strong tidal currents.
2. Global Market Trends
- Current Deployment: As of recent years, tidal turbines are being deployed in various locations, including the UK, France, and Canada. These regions have strong tidal resources and supportive regulatory frameworks.
- Future Growth: The market for tidal turbines is expected to grow as technology advances and costs decrease. Continued research and development, along with successful pilot projects, will drive wider adoption.
Future Prospects
The future of tidal turbine technology looks promising, with several key developments on the horizon:
- Technological Advancements: Innovations in turbine design, materials, and control systems are expected to improve efficiency and reduce costs.
- Increased Deployment: Expansion into new markets and larger-scale projects will drive the growth of the tidal energy sector.
- Environmental Impact: Ongoing research into the environmental effects of tidal turbines will ensure that they are deployed sustainably and with minimal impact on marine ecosystems.
Tidal turbines represent a significant advancement in renewable energy technology, offering a reliable and consistent source of power derived from tidal movements. With continued innovation and market development, tidal turbines have the potential to play a crucial role in the transition to a sustainable energy future. By understanding the various types of turbines, technological innovations, market dynamics, and future prospects, stakeholders can make informed decisions and contribute to the growth of this promising sector.
General Tips for Mechanical Engineering Assignments
Mechanical engineering assignments often require a deep understanding of complex concepts, meticulous attention to detail, and strong problem-solving skills. Here are some general tips to help you tackle mechanical engineering assignments effectively and achieve the best results.
1. Understand the Assignment Requirements
- Read the Prompt Carefully: Before starting, thoroughly read the assignment brief to understand what is required. Pay attention to specific questions, tasks, and any guidelines provided by your instructor.
- Clarify Doubts: If any part of the assignment is unclear, seek clarification from your instructor or peers. Ensuring you fully understand the requirements will help you focus your efforts effectively.
2. Plan and Organize Your Work
- Create an Outline: Organize your thoughts and structure your assignment by creating an outline. Break down the assignment into sections or tasks, such as introduction, methodology, analysis, and conclusion.
- Set Milestones: Establish deadlines for each part of the assignment to manage your time effectively. This will help you stay on track and ensure you complete the assignment on time.
3. Conduct Thorough Research
- Use Reliable Sources: Gather information from credible sources such as textbooks, peer-reviewed journals, and reputable websites. Ensure the sources are up-to-date and relevant to your topic.
- Take Detailed Notes: As you research, take detailed notes and keep track of important information, data, and references. This will make it easier to compile and reference your findings later.
4. Apply Engineering Principles
- Use Relevant Formulas and Theories: Apply appropriate engineering principles, theories, and formulas to solve problems. Ensure that you understand how to use these tools effectively and correctly.
- Show Your Work: Clearly demonstrate your problem-solving process. Include calculations, diagrams, and explanations to show how you arrived at your conclusions.
5. Write Clearly and Concisely
- Be Specific: Use precise language and avoid vague terms. Clearly define any technical terms or jargon used in your assignment.
- Structure Your Writing: Follow a logical structure in your writing, including an introduction, body, and conclusion. Use headings and subheadings to organize your content and make it easier to follow.
6. Review and Revise
- Proofread Your Work: Carefully review your assignment for errors in spelling, grammar, and formatting. Ensure that your writing is clear and coherent.
- Verify Calculations and Data: Double-check all calculations and data entries for accuracy. Mistakes in numerical data or calculations can lead to incorrect conclusions.
7. Use Visual Aids
- Include Diagrams and Charts: Where applicable, include diagrams, charts, and graphs to illustrate your points. Visual aids can help clarify complex concepts and make your assignment more engaging.
- Label Clearly: Ensure that all visual aids are clearly labeled and referenced in your text. Provide captions and explanations as needed.
8. Follow Formatting Guidelines
- Adhere to Guidelines: Follow any formatting and submission guidelines provided by your instructor. This may include specific requirements for font size, margins, referencing style, and document structure.
- Use Templates: If provided, use assignment templates or formats to ensure consistency with the required standards.
9. Seek Feedback
- Peer Review: Consider having a peer review your assignment before submission. They may provide valuable feedback and catch errors you might have missed.
- Consult Your Instructor: If possible, consult with your instructor or a tutor for feedback on drafts or to discuss any challenging aspects of the assignment.
10. Practice Problem-Solving Skills
- Work on Practice Problems: Regularly practice solving mechanical engineering problems to improve your skills and understanding. Use practice problems to familiarize yourself with different types of questions and solutions.
- Review Past Assignments: Review your previous assignments to identify areas for improvement and learn from past mistakes.
By following these tips, you can enhance your approach to mechanical engineering assignments, improve the quality of your work, and achieve better results. Effective planning, research, and problem-solving are key to successfully completing complex engineering tasks.
Conclusion
Successfully navigating mechanical engineering assignments requires a blend of strong technical knowledge, effective research, and clear communication. By following a structured approach, from understanding assignment requirements and conducting thorough research to applying engineering principles and reviewing your work, you can enhance the quality of your assignments and achieve better results.
Proper planning and organization are essential to manage your time effectively and ensure that you address all aspects of the assignment. Utilizing reliable sources, applying relevant theories and formulas, and presenting your findings clearly with appropriate visual aids will contribute to a comprehensive and well-rounded submission.
Additionally, adhering to formatting guidelines and seeking feedback from peers or instructors can further refine your work and prevent common mistakes. Regular practice and review of past assignments can also improve your problem-solving skills and overall performance.
By implementing these strategies, you can approach mechanical engineering assignments with confidence, effectively tackle complex problems, and ultimately succeed in your academic endeavors.