Advanced Techniques for Thermo-Mechanical Multiphysics Simulation with Abaqus
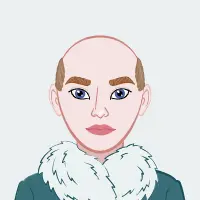
In the realm of advanced mechanical engineering, multiphysics simulations are essential for comprehending the intricate interactions between thermal and mechanical phenomena. These simulations become particularly valuable when analyzing components exposed to extreme conditions, which are common in fields like aerospace, automotive engineering, and other high-performance applications. By integrating multiple physical processes into a unified simulation framework, engineers can predict how components will behave under various operational stresses and environmental factors.
This blog delves into the methodology for conducting multiphysics simulations, with a particular focus on the coupled thermo-mechanical behavior of components subjected to high-temperature loading. Utilizing advanced simulation tools such as Abaqus, engineers can model complex scenarios where thermal and mechanical effects influence each other. For instance, understanding how a component like a turbine blade responds to high temperatures and cyclic loads requires a detailed approach that considers both thermal expansion and stress-induced deformation.
We will explore the key principles and techniques involved in setting up and executing these simulations. This includes defining material properties accurately, setting up the geometry, creating a high-quality mesh, and conducting both thermal and coupled thermo-mechanical analyses. Each step is crucial for obtaining reliable results that reflect real-world conditions. While our discussion will emphasize general methodologies, these insights are broadly applicable to a variety of engineering assignments involving multiphysics simulations.
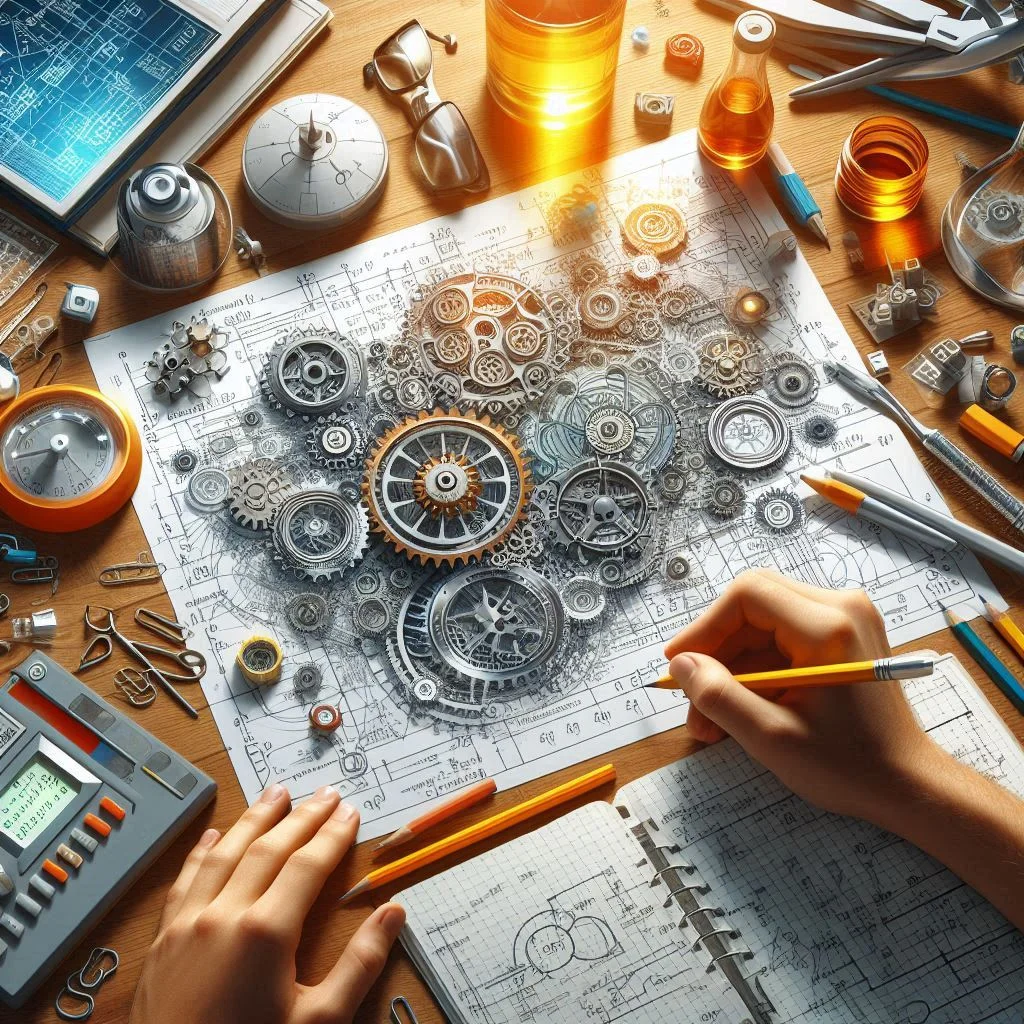
Whether you are working on simulating the behavior of aerospace components, automotive parts, or any other systems subjected to extreme conditions, understanding these advanced simulation techniques, including Abaqus assignment help, will enhance your ability to solve complex engineering problems.
Key Steps in Conducting a Multiphysics Simulation
Multiphysics simulations are integral to modern mechanical engineering, providing critical insights into the interplay between diverse physical phenomena. These simulations become indispensable in scenarios where components are exposed to complex, extreme conditions—such as high temperatures, varying mechanical loads, and intricate material behaviors. They are pivotal in industries such as aerospace, automotive, and energy, where understanding the combined effects of thermal and mechanical stresses is crucial for design optimization and performance reliability.
Achieving accurate and meaningful results from multiphysics simulations demands a meticulous and structured approach. Each step in the simulation process is vital to ensuring that the outcomes are reliable, reflective of real-world conditions, and actionable. Below, we delve into the key steps involved in conducting a multiphysics simulation, highlighting best practices and methodologies to guide you through the process and maximize the effectiveness of your analysis. For those seeking further assistance, mechanical engineering assignment help can provide valuable support in navigating complex simulations and optimizing your results.
Geometry and Preprocessing
The geometry and preprocessing phase lays the foundation for the entire simulation process. It begins with importing the 3D model of the component into your simulation software. These models typically originate from CAD systems or other design tools, and their accuracy directly impacts the quality of the simulation.
For complex components like turbine blades, it is often necessary to simplify the geometry. While simplification helps manage computational resources and reduces simulation time, it is crucial to retain critical features such as cooling channels, fillets, and areas prone to stress concentrations. These features are essential for accurate representation of the component’s behavior under operational conditions.
Preprocessing involves several key tasks:
- Defining Part Instances: Ensure that each component and its parts are correctly instantiated and positioned within the model. This includes defining boundary conditions that reflect the actual constraints and interactions of the component in its operating environment.
- Setting Up Symmetry: If applicable, use symmetry to reduce the computational cost. Symmetry can simplify the model without sacrificing accuracy by allowing you to simulate only a portion of the component and then mirror the results.
- Assembly and Configuration: Assemble the model and configure it to match the operational scenario. This involves setting up contacts, interactions, and boundary conditions accurately to reflect real-world conditions.
Proper geometry setup and preprocessing ensure that the model is both realistic and manageable, providing a solid base for the subsequent simulation steps.
Material Modeling
Material modeling is critical for ensuring that the simulation results are reflective of real-world behavior. This step involves defining the material properties that will be used in the simulation, including:
- Temperature-Dependent Properties: For applications involving high temperatures, such as turbine blades made from nickel-based superalloys, it is essential to define temperature-dependent properties. These include elastic modulus, thermal expansion coefficients, and yield strength, which vary with temperature and affect the component's performance.
- Creep and Phase Transformations: Implement models for complex material behaviors, such as creep and phase transformations. Creep modeling is important for understanding how materials deform over time under sustained loads and temperatures. Phase transformations, such as changes from austenitic to martensitic structures, can significantly impact material properties and behavior.
- Advanced Material Models: Depending on the complexity of the component and the accuracy required, you may need to incorporate advanced material models that account for non-linearities, anisotropy, and other factors affecting material behavior.
Accurate material modeling ensures that the simulations capture the nuanced behavior of the component under various conditions, leading to more reliable and actionable results.
Meshing
Meshing is the process of discretizing the geometry into finite elements, which allows the simulation software to perform calculations. Key considerations in meshing include:
- Mesh Quality: Generating a high-quality mesh is essential for accurate results. Use finer elements in regions with high stress concentrations or complex geometry to capture detailed behavior. For instance, in a turbine blade, finer mesh elements should be used around cooling channels and stress-prone areas.
- Element Types: Depending on the geometry and the analysis requirements, a combination of hexahedral and tetrahedral elements may be appropriate. Hexahedral elements are often used for regular geometries, while tetrahedral elements can be advantageous for complex shapes.
- Mesh Refinement Study: Conduct a mesh refinement study to evaluate the accuracy of the mesh. This involves comparing results from different mesh densities to find a balance between accuracy and computational cost. Refinement studies help ensure that the mesh is sufficient for capturing critical behaviors without unnecessary computational expense.
A well-designed mesh is crucial for obtaining accurate and reliable simulation results. It ensures that the finite element model can adequately represent the physical behavior of the component.
Thermal Analysis Setup
Before performing coupled analyses, it's essential to conduct a steady-state thermal analysis to determine the temperature distribution within the component. This involves:
- Modeling Heat Sources and Sinks: Accurately model heat fluxes, heat sources, and cooling mechanisms. For example, in a turbine blade, this would involve accounting for heat generated by combustion gases and cooling through internal channels.
- Thermal Boundary Conditions: Set up boundary conditions that reflect the actual thermal environment. This includes specifying convective and radiative heat transfer conditions, as well as initial temperature conditions.
The results from the thermal analysis provide the initial conditions for the thermo-mechanical analysis, ensuring that the thermal effects are accurately incorporated into the subsequent mechanical analysis.
Coupled Thermo-Mechanical Analysis
Once the thermal conditions are established, perform a coupled thermo-mechanical analysis to integrate the thermal and mechanical effects. This step involves:
- Applying Mechanical Loads: Apply relevant mechanical loads such as rotational forces, aerodynamic pressures, or other operational loads. Assess how these loads interact with the thermal expansion of the component.
- Evaluating Thermal Expansion: Analyze how thermal expansion affects mechanical stresses and how these stresses evolve under varying loads. This helps in understanding the component's performance under real-world conditions and identifying potential issues related to thermal and mechanical interactions.
A coupled approach provides a more comprehensive view of the component's behavior, simulating operational scenarios with greater accuracy.
Creep and Time-Dependent Analysis
Extend the simulation to include time-dependent phenomena such as creep to understand the long-term behavior of the component:
- Creep Modeling: Implement creep models to analyze how strain accumulates over time under sustained loads and temperatures. This involves using different creep models and material constants to study their effects on the component’s durability.
- Long-Term Performance: Evaluate how the material deforms and deteriorates over time. This provides insights into the component’s life expectancy and helps in assessing its suitability for long-term use.
Understanding creep behavior is essential for predicting the long-term performance and reliability of components subjected to prolonged operational conditions.
Post-Processing
After completing the simulations, use advanced post-processing tools to analyze the results:
- Temperature and Stress Analysis: Examine temperature distributions, stress concentrations, and creep strain accumulation. Use visualization tools to create detailed plots and animations, which can help illustrate how these factors evolve over time.
- Failure Zones: Identify potential failure zones and assess the component’s performance under the given conditions. Post-processing helps in interpreting the simulation data and drawing meaningful conclusions about the component’s reliability and performance.
Effective post-processing is crucial for making sense of the simulation results and guiding design improvements.
Reporting
The final step is preparing a comprehensive report that summarizes the entire simulation process:
- Modeling Process and Assumptions: Detail the modeling process, including the assumptions made, the methodologies used, and the setup of the simulation.
- Results and Implications: Summarize the simulation results and discuss their implications for component design. Highlight any potential design improvements or performance enhancements.
- Recommendations for Future Work: Provide recommendations for future work, such as exploring advanced material models, validating results through experimental methods, or refining simulation techniques.
A well-documented report serves as a valuable resource for understanding the results, making informed design decisions, and guiding future analysis efforts.
Conclusion
Multiphysics simulations are indispensable tools in the modern engineering toolkit, offering profound insights into the behavior of components subjected to complex and multifaceted conditions. By integrating thermal and mechanical analyses, these simulations provide a holistic view of how engineering components respond to a range of operational stresses and environmental factors. This comprehensive approach is critical for predicting performance, optimizing designs, and ensuring reliability in demanding applications.
The outlined key steps—geometry and preprocessing, material modeling, meshing, thermal analysis setup, coupled thermo-mechanical analysis, creep and time-dependent analysis, post-processing, and reporting—form the foundation of an effective simulation process. Each step is crucial in ensuring that the simulation accurately reflects real-world conditions and delivers meaningful insights. Properly executed simulations enable engineers to explore and understand intricate interactions between different physical phenomena, leading to better-informed design decisions and enhanced performance.
- Geometry and Preprocessing: Accurate geometry import and preprocessing are fundamental for setting up realistic models. Properly simplifying the geometry while retaining essential features ensures that the model is both computationally manageable and representative of the actual component.
- Material Modeling: Defining material properties with precision, including temperature-dependent behaviors and complex phenomena like creep and phase transformations, is essential for capturing the true performance of materials under various conditions. This detailed modeling helps in predicting how materials will behave in high-stress and high-temperature environments.
- Meshing: Creating a high-quality mesh that balances accuracy and computational efficiency is crucial for reliable results. Fine meshing in critical areas helps capture detailed stress and strain distributions, while mesh refinement studies ensure that the results are robust and accurate.
- Thermal Analysis Setup: Conducting a thorough thermal analysis provides critical data on temperature distributions, which influence mechanical behavior. Accurate thermal modeling ensures that the initial conditions for subsequent analyses reflect real-world thermal effects, enhancing overall simulation reliability.
- Coupled Thermo-Mechanical Analysis: Integrating thermal and mechanical analyses allows for a comprehensive assessment of component performance. This approach simulates real-world conditions more effectively, revealing how thermal variations affect mechanical stresses and vice versa.
- Creep and Time-Dependent Analysis: Including time-dependent phenomena such as creep is vital for understanding long-term performance and durability. This analysis helps in evaluating how materials will deform and degrade over time, providing insights into component lifespan and suitability for extended use.
- Post-Processing: Effective post-processing involves analyzing and visualizing simulation results to draw meaningful conclusions. By examining temperature distributions, stress concentrations, and creep strain, engineers can identify potential failure zones and make informed decisions about design improvements.
- Reporting: A well-prepared report consolidates the modeling process, simulation results, and their implications. This comprehensive documentation serves as a valuable resource for understanding findings, guiding design enhancements, and supporting future analysis efforts.
Accurate multiphysics simulations not only enhance component design and performance but also drive innovation and efficiency in engineering practices. Mastery of these techniques empowers engineers to tackle complex challenges with confidence, leading to optimized designs and improved reliability of critical components. For those navigating intricate engineering problems, additional resources and expert support are available to provide further assistance with multiphysics simulations and related engineering challenges.
Embracing these practices ensures that engineering solutions are well-informed, robust, and capable of meeting the demands of modern applications. By leveraging advanced simulation techniques and staying abreast of evolving methodologies, engineers can continue to push the boundaries of innovation and achieve exceptional results in their projects.