Integrating Biomolecular Feedback Systems in Mechanical Engineering
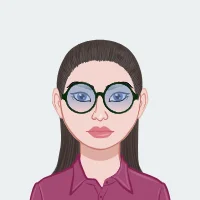
In the realm of mechanical engineering, biomolecular feedback systems present a fascinating intersection of biological complexity and engineering analysis. These systems, comprising intricate networks of biochemical reactions and regulatory feedback loops, offer compelling challenges that demand a systematic and analytical approach to problem-solving. Understanding these dynamics involves delving into hypothetical scenarios that mirror real-world assignments, emphasizing not just theoretical concepts but also practical methodologies essential for navigating the complexities of biomolecular interactions. By focusing on foundational principles rather than prescriptive solutions, students can cultivate a deep understanding of how to analyze equilibria, assess stability, and model dynamic behaviors within the context of mechanical engineering applications. This holistic approach not only enhances academic learning but also prepares students to confidently tackle and solve their bioengineering assignment.
Assignment Overview
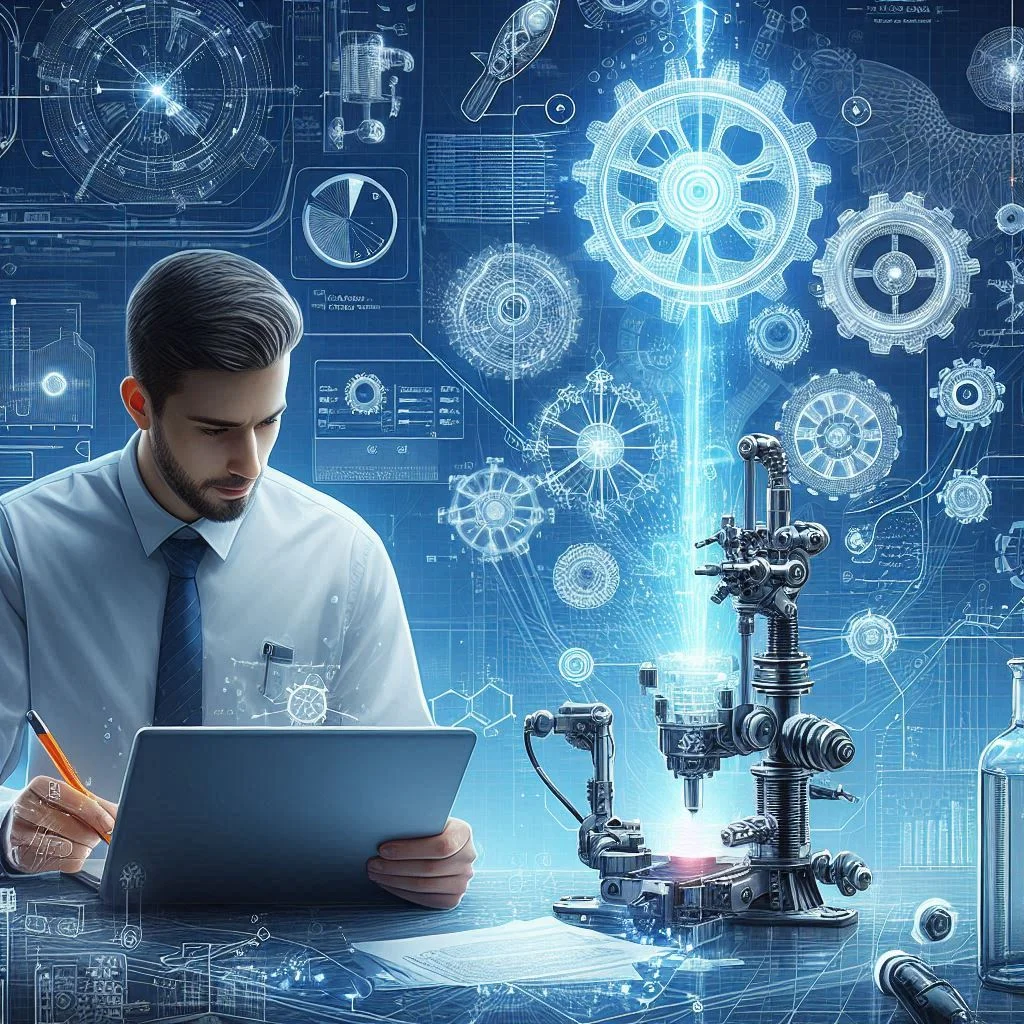
Imagine immersing yourself in a rigorous assignment that explores the intricacies of biomolecular feedback systems within the context of mechanical engineering. This hypothetical scenario offers a stimulating journey into understanding dynamic networks of biochemical reactions and regulatory mechanisms. By delving into this complex terrain, you'll gain invaluable insights into the analytical methodologies required to decipher equilibrium states, assess stability under varying parameters, and model dynamic behaviors with precision. This comprehensive exploration not only enhances theoretical understanding but also hones practical problem-solving skills essential for navigating the multifaceted challenges of biomolecular interactions within mechanical engineering.
Problem 1: Analyzing Equilibria and Stability
Consider diving into the detailed analysis of equilibria and stability within biomolecular feedback systems, a cornerstone of mechanical engineering assignments. This problem prompts you to explore the dynamic interplay of biochemical reactions and regulatory mechanisms. By methodically examining how changes in parameters such as α, K, γ, δ, and n influence equilibrium states, you'll uncover the fundamental principles governing stability. Utilizing sophisticated tools like nullcline analysis and linearization techniques, you'll not only determine the number of equilibria but also scrutinize their stability under varying conditions. This rigorous approach prepares you to navigate complex scenarios, equipping you with essential skills to tackle similar challenges in mechanical engineering with confidence and precision.
1. Determining Equilibria:
Begin your exploration by delving into the intricate ways in which alterations in parameters such as α, K, γ, δ, and n impact the equilibrium states within the biomolecular feedback system. These parameters not only define the biochemical reactions and regulatory mechanisms but also influence the system's overall stability and behavior. By systematically varying these parameters and meticulously analyzing their effects, you gain a deeper understanding of how equilibrium points emerge and evolve under different physiological or environmental conditions. This foundational investigation forms the bedrock for further detailed analysis of stability and dynamic interactions within the system, providing essential insights for engineering solutions and optimizations.
2. Stability Analysis:
Embark on a rigorous analysis employing sophisticated tools like nullcline analysis and linearization techniques to scrutinize the stability of identified equilibria. Nullcline analysis offers a visual representation of how the rates of change of key biochemical species intersect, revealing stable, unstable, and oscillatory states within the system. Linearization around equilibrium points enables a quantitative assessment of stability through eigenvalue analysis or phase plane methods, elucidating the system's response to perturbations. This analytical depth not only allows you to predict and interpret system behaviors under varying parameter regimes but also informs strategic interventions to enhance stability and performance in mechanical engineering applications.
3. Dynamic Interactions:
Navigate the intricate web of dynamic interactions within the biochemical network, uncovering how regulatory mechanisms modulate equilibrium stability and system behavior. Explore feedback loops, feedforward mechanisms, and nonlinear interactions that govern the system's response to stimuli and maintain homeostasis. By dissecting these interactions, you gain insights into how regulatory elements amplify or attenuate biochemical signals, influencing the system's robustness and adaptability. This holistic understanding not only enhances your theoretical grasp of biomolecular dynamics but also empowers you to design targeted interventions that optimize system performance and resilience in mechanical engineering contexts.
Through this structured and comprehensive approach, you develop a robust foundation in analyzing biomolecular systems, equipped with the clarity, depth, and expertise needed to navigate and solve nuanced challenges in mechanical engineering assignments effectively. These skills are essential for tackling real-world problems and advancing innovative solutions that integrate biological complexity with engineering precision.
Problem 2: Quasi-Steady State Assumption (QSSA)
In the dynamic field of mechanical engineering, grasping the intricacies of the Quasi-Steady State Assumption (QSSA) is indispensable for unraveling the dynamics of biomolecular feedback systems. This problem immerses you in an in-depth exploration of how mRNA dynamics shape system behavior and stability, providing a structured approach to untangle complexities:
1. Introduction to QSSA:
Begin by establishing a robust theoretical foundation on QSSA, a methodological tool pivotal in simplifying the rapid dynamics of mRNA within intricate biochemical networks. This assumption allows for a focused analysis on steady-state conditions, bypassing transient fluctuations to streamline the understanding of system behavior. By embracing QSSA, you gain clarity on the essential dynamics governing biomolecular systems, enabling precise analysis and prediction of system responses under various conditions.
2. Model Development:
Forge ahead with the development of a comprehensive model that integrates the QSSA framework. Construct equations that prioritize the equilibrium conditions of critical biochemical species, while incorporating the steady-state assumption for mRNA dynamics. This model serves as a foundational framework to scrutinize how system stability evolves with changes in parameters and environmental conditions. By meticulously defining these dynamics, you gain insights into the resilience and adaptability of biomolecular systems, pivotal for engineering robust solutions.
3. Stability Analysis:
Harness advanced stability analysis techniques to rigorously assess the robustness of equilibrium points within your QSSA model. Utilize methods such as eigenvalue analysis or phase plane analysis to delve into how variations in parameters—such as α, K, γ, δ, and n—affect system behavior and stability. This analytical depth unveils critical insights into the system's response mechanisms, guiding strategic interventions and optimizations in mechanical engineering applications.
4. Comparative Study:
Conduct a comprehensive comparative study between outcomes derived from your QSSA model and those from a more detailed, non-QSSA model (as explored in Problem 1). Highlight the advantages and limitations of the QSSA approach in accurately predicting system dynamics and stability. Emphasize its efficacy in simplifying complex biochemical networks while acknowledging nuances that a detailed model captures. This comparative analysis sharpens your ability to leverage QSSA effectively in real-world scenarios, bridging theoretical concepts with pragmatic engineering solutions.
By immersing yourself deeply in these sub-parts, you cultivate a nuanced understanding of how QSSA enhances your prowess in modeling and analyzing biomolecular feedback systems. This knowledge equips you with indispensable skills to navigate and resolve intricate challenges in mechanical engineering assignments, translating theoretical insights into actionable strategies for innovation and problem-solving.
Problem 3: Integrating mRNA Dynamics
In the expansive domain of mechanical engineering, effectively integrating mRNA dynamics within biomolecular feedback systems represents a significant challenge, necessitating a meticulous and comprehensive approach to analysis and modeling. This problem immerses you in the intricate dynamics of how mRNA influences system behavior and stability, offering a structured exploration to uncover profound insights:
1. Conceptual Framework:
Establish a robust conceptual framework that intricately weaves together the dynamics of mRNA synthesis, degradation, and regulatory mechanisms within the biochemical network. Consider the nonlinear interactions and feedback loops that govern mRNA levels and their impact on biochemical pathways. This foundational understanding lays the groundwork for developing a nuanced model that captures both transient fluctuations and long-term behaviors influenced by mRNA dynamics.
2. Model Development:
Progress to develop an advanced model that intricately incorporates the dynamic behavior of mRNA. Construct differential equations that meticulously account for mRNA synthesis rates, degradation kinetics, and their intricate interactions with other biochemical species. Integrate regulatory pathways and feedback mechanisms to simulate how changes in mRNA expression levels propagate through the system, affecting equilibrium states and overall system stability over time.
3. Steady-State and Transient Analysis:
Undertake rigorous steady-state and transient analyses to delve into how the system responds to external perturbations and varying environmental conditions. Employ numerical methods or sophisticated simulation techniques to predict the temporal evolution of mRNA levels and their profound impact on biochemical pathways. Contrast transient responses with steady-state predictions to glean insights into system adaptability, resilience, and capacity for homeostasis.
4. Parameter Sensitivity and Optimization:
Conduct comprehensive sensitivity analyses to discern the sensitivity of system behaviors to fluctuations in critical parameters such as mRNA synthesis rates, degradation constants, and regulatory thresholds. Identify pivotal parameters that exert significant influence on system performance and explore optimization strategies aimed at fortifying system robustness, efficiency, and overall functionality in dynamic mechanical engineering contexts.
5. Comparative Study with QSSA:
Engage in a comparative study to juxtapose outcomes derived from the detailed mRNA-integrated model against those derived from simplified models utilizing the Quasi-Steady State Assumption (QSSA), as explored in Problem 2. Illuminate disparities in predictive accuracy, computational efficiency, and practical applicability between these modeling approaches. Foster a discourse on the trade-offs and pragmatic considerations involved in selecting between detailed kinetic models and simplified assumptions for informing engineering decisions and facilitating innovative advancements in real-world applications.
By immersing deeply into these sub-parts, you will cultivate a comprehensive and profound understanding of how integrating mRNA dynamics enhances the fidelity and utility of biomolecular feedback system models in mechanical engineering. This knowledge empowers you with analytical prowess, strategic insights, and practical acumen to navigate complex challenges, drive innovation in system design, and optimize performance across diverse mechanical engineering domains.
Conclusion
In conclusion, the study of integrating mRNA dynamics within biomolecular feedback systems represents a crucial frontier in mechanical engineering, where understanding the nuances of biochemical interactions is essential for advancing system design and optimization. Throughout our exploration, we have delved deeply into the complexities of mRNA dynamics, emphasizing their profound influence on system behavior and stability.
Our journey began with establishing a robust conceptual framework that elucidated the intricate relationships between mRNA synthesis, degradation kinetics, and regulatory mechanisms within biochemical networks. This foundational understanding provided the basis for developing sophisticated models capable of capturing the dynamic evolution of mRNA levels under varying conditions.
Through rigorous analysis techniques, including steady-state and transient analyses, we uncovered how the system responds dynamically to external stimuli and internal perturbations. These analyses not only highlighted the system's adaptive capabilities but also underscored the importance of considering transient effects in addition to steady-state behaviors.
Sensitivity analyses further enhanced our insights by identifying critical parameters that significantly impact system performance. This understanding is pivotal for optimizing system design, improving robustness, and ensuring reliable operation in practical engineering applications.
The comparative study with the Quasi-Steady State Assumption (QSSA) provided valuable insights into the trade-offs between model complexity and computational efficiency. While QSSA offers simplifications that facilitate rapid analysis, our detailed kinetic models demonstrated superior predictive accuracy, particularly in capturing transient dynamics and complex interactions within biomolecular networks.
In essence, this exploration equips mechanical engineers with advanced tools and methodologies to tackle the intricacies of biomolecular systems effectively. By bridging theoretical understanding with practical applications, engineers can innovate with confidence, designing systems that are not only robust and efficient but also adaptable to changing environmental conditions.
Looking forward, continued research in integrating mRNA dynamics will pave the way for groundbreaking advancements in mechanical engineering. By leveraging these insights, engineers can drive transformative solutions that integrate biological complexity with engineering precision, shaping the future of biomedical devices, biotechnology, and sustainable engineering practices.
This journey underscores the interdisciplinary nature of modern engineering, where insights from biology and chemistry converge with mechanical principles to forge new frontiers in technology and innovation. As we continue to unravel the mysteries of biomolecular systems, the opportunities for impactful advancements in mechanical engineering are limitless.