Breaking Down the Basics of Mechanical Vibrations in Engineering Assignments
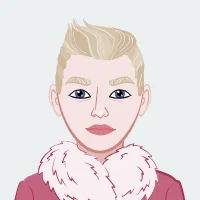
Mechanical vibrations play a pivotal role in the realm of engineering, serving as a fundamental and intricate aspect that shapes the design, analysis, and functionality of various mechanical systems. At its core, mechanical vibrations encompass oscillatory motion and dynamic behavior, making them indispensable in understanding and optimizing the performance of structures and machinery. The importance of comprehending mechanical vibrations lies in their pervasive influence across diverse engineering disciplines, from civil and aerospace to automotive and industrial engineering. If you require help with your mechanical engineering assignment, our insights into mechanical vibrations can provide valuable assistance in mastering this essential aspect of engineering.
In real-world applications, the impact of mechanical vibrations is ubiquitous. Consider a suspension system in an automobile, where vibrations directly influence ride comfort, handling, and overall vehicle performance. In civil engineering, the behavior of structures subjected to seismic forces involves intricate vibrational analysis to ensure resilience and safety. Furthermore, the aerospace industry relies on a profound understanding of mechanical vibrations to design aircraft that withstand the complex forces encountered during flight.
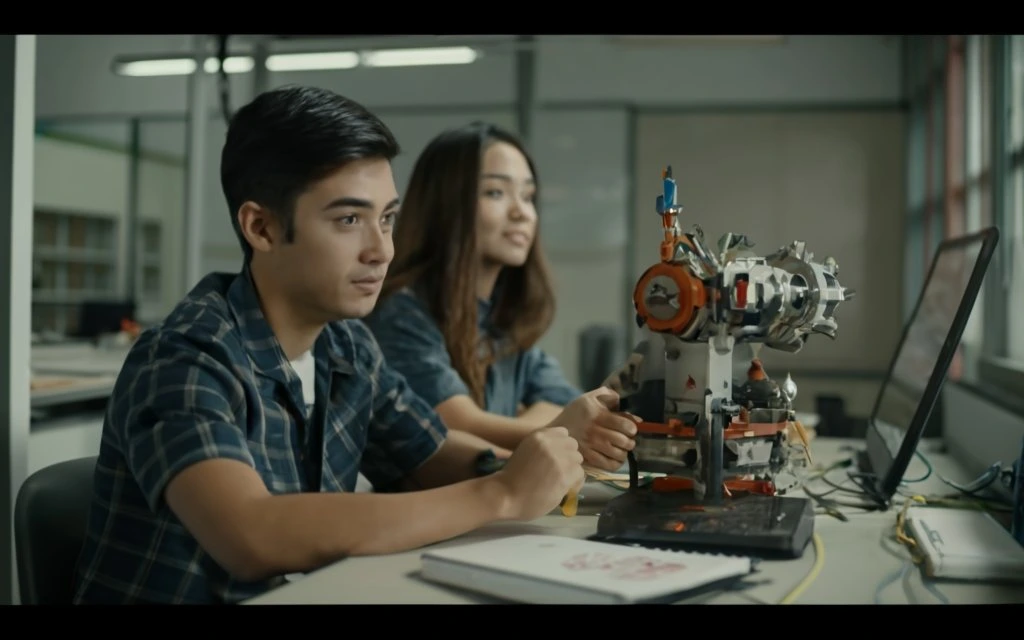
Academically, the study of mechanical vibrations is not merely a theoretical pursuit but an essential component of engineering education. Students grappling with assignments related to mechanical vibrations delve into the intricacies of mathematical models, equations, and analytical methods that form the basis for predicting and controlling vibrational phenomena. This academic exploration prepares future engineers to tackle real-world challenges by instilling problem-solving skills and a deep understanding of the dynamic behavior of mechanical systems.
In engineering assignments, students are often tasked with applying the principles of mechanical vibrations to practical scenarios, mirroring the challenges they will encounter in their professional careers. Whether designing a structure resilient to vibrations or optimizing machinery for smoother operation, these assignments bridge the gap between theoretical knowledge and its practical application. Students gain not only a theoretical foundation in mechanical vibrations but also the ability to translate this knowledge into effective solutions for real-world engineering problems.
As engineering continues to advance and embrace innovative technologies, the role of mechanical vibrations in shaping the performance and reliability of systems becomes increasingly crucial. Through a nuanced understanding of mechanical vibrations, engineers can optimize designs, enhance structural integrity, and ensure the functionality of a wide array of mechanical systems, ultimately contributing to the advancement of technology and the improvement of everyday life. Therefore, the study of mechanical vibrations stands as an indispensable pillar in the education and practice of mechanical engineering, forging a link between theoretical principles and their transformative application in the physical world.
Understanding Mechanical Vibrations
Mechanical vibrations, in the realm of engineering, refer to the oscillatory motion of mechanical systems around an equilibrium point. These vibrations are caused by dynamic forces acting on a structure or a component, leading to periodic fluctuations in position. The significance of understanding mechanical vibrations lies in their pervasive influence on the performance, safety, and longevity of mechanical systems. Engineers must grapple with the complexities of vibrations to design structures and machinery that not only meet functional requirements but also withstand the dynamic forces they encounter in real-world applications.
There are several types of mechanical vibrations, each with distinct characteristics and implications for engineering applications. Free vibrations occur when a mechanical system is disturbed from its equilibrium position and allowed to oscillate freely without external influences. These vibrations are entirely dependent on the system's inherent properties and initial conditions. In contrast, forced vibrations result from the application of external forces or excitations to a system, imposing a specific frequency of oscillation. This type of vibration is common in machinery subjected to periodic loads, and engineers must carefully analyze and control forced vibrations to prevent structural damage or performance degradation.
Damped and undamped vibrations represent another critical distinction. Damped vibrations occur when energy is dissipated from the vibrating system, leading to a gradual decay in amplitude over time. Damping is crucial in engineering applications to mitigate the risk of resonance and prevent excessive oscillations that could compromise structural integrity. Undamped vibrations, on the other hand, persist indefinitely in the absence of external influences or energy dissipation. While undamped vibrations are theoretically simpler to analyze, they can pose challenges in real-world applications due to their potential for amplification and resonance.
In mechanical engineering, the ability to analyze and control vibrations is paramount. Engineers must navigate the intricacies of mathematical models, equations, and sophisticated analytical techniques to predict and mitigate the effects of vibrations in diverse applications. Whether designing a skyscraper resistant to seismic vibrations, optimizing an aircraft's wing to withstand turbulent airflows, or ensuring the smooth operation of rotating machinery, a profound understanding of mechanical vibrations is indispensable. By mastering the principles of free and forced vibrations, as well as damped and undamped vibrations, engineers empower themselves to create robust and reliable systems that can thrive in the dynamic and challenging environments of the modern world.
Mathematical Concepts Behind Vibrations
The mathematical principles governing mechanical vibrations are fundamental to understanding the dynamic behavior of structures and mechanical systems. At the heart of this understanding is the utilization of differential equations, which describe how forces, mass, and displacement interact within a vibrating system. These equations of motion serve as a cornerstone in modeling and predicting the oscillatory patterns exhibited by mechanical systems.
A key concept in mechanical vibrations is simple harmonic motion (SHM), which provides a fundamental framework for analyzing linear, undamped systems. In the context of SHM, the motion of a system follows a sinusoidal pattern, reflecting the characteristic back-and-forth oscillations that define mechanical vibrations. This mathematical representation allows engineers to gain insights into the dynamic nature of the system and predict its behavior over time.
When damping is introduced into the system to account for energy dissipation, the mathematical formulation becomes more intricate. The presence of damping forces, represented by a damping coefficient, leads to damped harmonic motion. This modification acknowledges that in real-world scenarios, mechanical systems experience resistance that gradually reduces the amplitude of vibrations over time. Understanding these damped vibrations is crucial for engineers as it influences the system's response to external forces and its overall stability.
In addition to differential equations, engineers employ various formulas to quantify and analyze mechanical vibrations. These may include expressions for natural frequency, which characterizes the inherent oscillation frequency of a system, or formulas that relate the amplitude of vibrations to external forces and system parameters. These mathematical tools empower engineers to design structures and machinery that not only meet functional requirements but also possess the necessary resilience to withstand dynamic forces encountered in real-world applications.
In summary, the mathematical principles underpinning mechanical vibrations provide a comprehensive framework for engineers to analyze and predict the dynamic behavior of systems. From simple harmonic motion to damped vibrations, these mathematical tools enable engineers to design and optimize structures, ensuring they can withstand the challenges posed by dynamic forces in various engineering applications.
H2: Practical Applications in Engineering Assignments
The understanding of mechanical vibrations plays a pivotal role in engineering assignments, where students are tasked with applying theoretical knowledge to practical scenarios, mirroring the challenges they will encounter in their professional careers. One critical application is in the design and analysis of structures subjected to dynamic loads, such as buildings, bridges, and industrial machinery. In assignments focusing on structural engineering, students must employ their knowledge of mechanical vibrations to predict and mitigate the effects of forces like wind, earthquakes, or machinery-induced vibrations. For instance, designing a skyscraper that can withstand seismic activity necessitates a thorough understanding of the building's natural frequencies and the potential resonance effects that may arise during an earthquake.
In the realm of automotive engineering, assignments often revolve around optimizing vehicle components for a smooth and comfortable ride. Here, mechanical vibrations are of paramount importance as they directly impact the ride comfort, handling, and overall performance of automobiles. Students may be tasked with designing suspension systems that effectively dampen vibrations transmitted from uneven road surfaces or addressing resonance issues in components like the steering system. The ability to analyze and control vibrations is crucial in ensuring that the vehicle not only meets safety standards but also provides a comfortable and stable ride for passengers.
Assignments related to machinery and mechanical systems also frequently incorporate the principles of mechanical vibrations. For instance, in assignments focused on rotating machinery like engines or turbines, students must apply their knowledge to optimize the components for minimal vibrations, ensuring smooth operation and longevity. They may need to analyze the effects of unbalanced loads, misalignments, or other dynamic forces that could lead to excessive vibrations and potential system failures.
Another significant area is in the field of aerospace engineering, where knowledge of mechanical vibrations is indispensable for designing aircraft that can withstand the complex forces encountered during flight. Students may be tasked with analyzing the vibrations induced by aerodynamic forces, engine operation, or turbulence, and devising solutions to minimize their impact on the structural integrity and performance of the aircraft.
In essence, the understanding of mechanical vibrations in engineering assignments extends across various disciplines, from civil and automotive to machinery and aerospace engineering. The application of this knowledge is evident in the optimization of structures and systems to meet performance criteria, ensuring that engineers are well-equipped to tackle real-world challenges in their future professional endeavors.
Tools and Techniques for Analyzing Vibrations
Analyzing mechanical vibrations in engineering involves the use of various tools and techniques to comprehend and mitigate the effects of dynamic forces on structures and machinery. One common tool is the vibration analyzer, a portable device that measures and records vibrations in real-time. Vibration analyzers help engineers identify the frequencies and amplitudes of vibrations, enabling them to pinpoint potential issues such as resonance or imbalance. Another widely used tool is the accelerometer, a sensor that measures acceleration and is essential for collecting data on vibrational movements. Accelerometers are often employed in conjunction with data acquisition systems to monitor and analyze vibrations in different engineering applications.
Finite Element Analysis (FEA) is a powerful numerical technique extensively used in engineering assignments to model and simulate mechanical vibrations. FEA breaks down complex structures into smaller, manageable elements, allowing engineers to analyze their dynamic behavior under various conditions. This method provides insights into the natural frequencies, mode shapes, and stress distributions within a structure, aiding in the design process and identifying potential areas of concern related to vibrations.
In the realm of software, MATLAB is a prevalent tool for conducting vibrational analysis. It offers a comprehensive suite of functions and toolboxes specifically designed for signal processing and vibration analysis. Engineers use MATLAB to solve complex mathematical equations, simulate mechanical systems, and visualize vibrational responses. Additionally, ANSYS, a finite element analysis software, is widely utilized for simulating and optimizing mechanical vibrations. ANSYS enables engineers to model complex structures and predict their dynamic behavior, aiding in the identification of potential design flaws or areas susceptible to vibrational issues.
Multibody Dynamics (MBD) simulations are another valuable technique used to analyze mechanical vibrations. MBD software, such as Adams or Simpack, allows engineers to model the motion and interactions of interconnected rigid or flexible bodies. This approach is particularly beneficial for studying the dynamic behavior of complex mechanisms like vehicle suspension systems or machinery with moving parts.
In engineering assignments, students often employ these tools and techniques to analyze and solve problems related to mechanical vibrations. Tasks may include predicting natural frequencies, determining critical damping ratios, or optimizing system components to minimize vibrational effects. The integration of theoretical knowledge with practical applications using these tools equips students with the skills necessary to address real-world challenges in fields such as structural design, automotive engineering, and machinery optimization. Ultimately, the combination of analytical tools and software facilitates a comprehensive understanding of mechanical vibrations and empowers engineers to design resilient and efficient systems.
Tips for Solving Mechanical Vibration Assignments
Tackling assignments related to mechanical vibrations can be both intellectually rewarding and challenging for students. Here are some practical tips to guide students through these assignments, along with insights into common challenges and strategies to overcome them.
Develop a Solid Foundation:
Before delving into assignments, ensure a strong understanding of fundamental concepts such as simple harmonic motion, damping, and resonance. Familiarity with mathematical tools like differential equations and Fourier analysis will lay a robust foundation for approaching vibrational problems.
Practice with Real-world Examples:
Apply theoretical knowledge to real-world examples. Explore how mechanical vibrations manifest in everyday situations, from the sway of a suspension bridge to the vibrations in a washing machine. This practical application enhances comprehension and prepares students for more complex assignments.
Master Analytical Tools:
Proficiency in using analytical tools such as MATLAB for signal processing and finite element analysis (FEA) software is crucial. Invest time in learning these tools as they are indispensable for simulating and analyzing mechanical vibrations, providing valuable insights into system behavior.
Break Down Complex Systems:
When faced with complex systems, break them down into simpler components. Analyze each component individually before integrating them to understand how they interact. This step-by-step approach aids in solving intricate vibrational problems more effectively.
Identify and Address Common Challenges:
Common challenges in mechanical vibrations assignments include difficulties in solving differential equations, understanding the effects of damping, and predicting natural frequencies accurately. Address these challenges by seeking additional resources, collaborating with peers, and consulting professors or online tutorials.
Experiment with Numerical Methods:
Employ numerical methods to validate theoretical results. Finite difference methods or numerical integration techniques can be valuable for approximating solutions, especially when analytical solutions are challenging to obtain. This hands-on approach enhances problem-solving skills.
Consider Boundary Conditions:
Pay careful attention to boundary conditions in vibrational analysis. Incorrectly specified constraints can lead to inaccurate results. Ensure a thorough understanding of how different boundary conditions affect the vibrational behavior of structures or systems.
Embrace Iterative Problem-solving:
Mechanical vibrations assignments often require an iterative problem-solving approach. Don't hesitate to revisit and refine solutions. Iterative processes help in improving accuracy and gaining a deeper understanding of the problem at hand.
Seek Support and Collaborate:
Engage with classmates, form study groups, or seek guidance from professors. Collaborative learning enhances the understanding of diverse approaches to solving vibrational problems and provides valuable insights.
Time Management:
Mechanical vibrations assignments can be time-consuming. Plan your time wisely, start early, and break down the assignment into manageable tasks. This approach helps in avoiding last-minute stress and ensures a more thorough understanding of the material.
By following these practical tips, students can navigate the challenges of mechanical vibrations assignments more effectively and develop the skills needed for successful problem-solving in the field of mechanical engineering.
Conclusion:
In the blog post, "Breaking Down the Basics of Mechanical Vibrations in Engineering Assignments," we explored the fundamental aspects of mechanical vibrations and their crucial role in the field of engineering. The significance of understanding mechanical vibrations in real-world applications and academic assignments was highlighted, emphasizing its impact on structural design, automotive engineering, machinery optimization, and aerospace technology. The mathematical principles governing mechanical vibrations were discussed, covering key equations such as the second-order linear differential equation and the simple harmonic motion equation, as well as the distinctions between free and forced vibrations, and damped and undamped vibrations.
The application of this theoretical knowledge in engineering assignments was illustrated across various disciplines. Examples included the design of earthquake-resistant structures, optimization of vehicle suspension systems for a smoother ride, and the analysis of rotating machinery to prevent excessive vibrations. The blog delved into common tools and techniques used to analyze mechanical vibrations, including vibration analyzers, accelerometers, Finite Element Analysis (FEA), MATLAB, and Multibody Dynamics (MBD) simulations. The integration of these tools empowers engineers to model, simulate, and predict the dynamic behavior of mechanical systems accurately.
For students tackling assignments related to mechanical vibrations, practical tips were offered to enhance their understanding and problem-solving skills. These tips encompassed developing a strong foundational knowledge, practicing with real-world examples, mastering analytical tools, breaking down complex systems, experimenting with numerical methods, and considering boundary conditions. Additionally, the importance of time management and collaborative learning was emphasized to overcome common challenges associated with solving differential equations, understanding damping effects, and predicting natural frequencies accurately.
In conclusion, the blog post aimed to provide a comprehensive overview of mechanical vibrations in engineering assignments, offering insights into theoretical principles, practical applications, and problem-solving techniques. The encouragement for students to delve deeper into the subject and seek assistance if needed was underscored. Delving deeper into the subject allows students to gain a more profound understanding of mechanical vibrations, equipping them with the skills necessary for success in their academic pursuits and future engineering careers. Seeking assistance when faced with challenges fosters a supportive learning environment, ensuring that students can overcome obstacles and master the complexities of mechanical vibrations in their educational journey.