Navigating Bioengineering Assignments: Insights into Microscale Engineering Projects
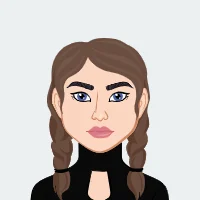
Microscale engineering in bioengineering is an exciting and rapidly evolving field that combines principles of engineering with biological sciences. This interdisciplinary approach is critical for developing innovative solutions for medical diagnostics, therapeutic devices, and biological research. By integrating advanced microfabrication techniques with biological principles, engineers can design microscale devices that offer unprecedented levels of precision and functionality. These devices are pivotal in areas such as point-of-care diagnostics, where rapid and accurate analysis of biological samples can significantly improve patient outcomes.
Understanding the fundamental concepts and applications of microscale engineering is essential for navigating the complexities of modern mechanical engineering assignments and projects. It involves mastering intricate processes such as microfluidics, where minute volumes of fluids are manipulated to perform complex biochemical assays. Moreover, proficiency in material selection and fabrication methods like photolithography and soft lithography enables engineers to tailor devices to specific biomedical applications, ensuring optimal performance and reliability.
.webp)
Moreover, as bioengineering continues to push the boundaries of what is possible in healthcare and scientific research, the demand for skilled professionals versed in microscale engineering is expected to grow. This field not only demands technical expertise but also fosters creativity and problem-solving skills to address challenges such as enhancing device sensitivity, improving biocompatibility, and scaling up production for clinical use.
In conclusion, mastering microscale engineering in bioengineering opens up a world of opportunities to contribute to groundbreaking advancements that have the potential to transform healthcare and biological research. Whether you are analyzing the effectiveness of novel microchip technologies or designing next-generation therapeutic devices, a comprehensive understanding of microscale engineering principles will empower you to make meaningful contributions to this dynamic field. For expert guidance in exploring these realms and tackling assignments in bioengineering engineering, consider accessing reliable bioengineering assignment help resources to enhance your learning journey.
Key Concepts and Applications in Microscale Engineering in Bioengineering
Microscale engineering in bioengineering represents a dynamic intersection of engineering principles and biological sciences, driving innovation in medical diagnostics, therapeutic interventions, and biological research. This field harnesses the unique capabilities of microfabrication techniques to create sophisticated devices and systems that operate at scales where biological processes unfold, offering unprecedented precision and functionality.
Key Concepts:
- Microfluidics and Lab-on-a-Chip Technology: Microfluidics involves the precise manipulation of fluids at the microscale level, facilitating tasks such as sample preparation, chemical analysis, and cell manipulation within miniaturized systems. Lab-on-a-chip devices integrate multiple laboratory functions onto a single chip, enabling portable and rapid diagnostic solutions.
- Microscale Sensors and Actuators: Sensors and actuators at the microscale are essential for detecting and responding to biological signals with high sensitivity and specificity. These components include optical sensors, electrochemical sensors, and microvalves, which play crucial roles in biomedical devices for monitoring biomarkers and controlling fluid flows.
- Materials and Fabrication Techniques: Selecting appropriate materials and fabrication techniques is critical in designing microscale bioengineering devices. Materials such as silicon, polymers, and glass offer distinct advantages in terms of biocompatibility, mechanical properties, and optical transparency. Fabrication methods like photolithography and 3D printing enable precise control over device geometries and functionalities.
Applications:
- Biomedical Diagnostics: Microscale engineering enables the development of advanced diagnostic tools that provide rapid and accurate analysis of biological samples. These tools are used in point-of-care testing, disease monitoring, and personalized medicine applications, enhancing healthcare delivery and patient outcomes.
- Therapeutic Devices: Bioengineered microdevices are used in targeted drug delivery systems, tissue engineering scaffolds, and neural interfaces. These devices facilitate precise control over drug release kinetics, cellular interactions, and neural signal modulation, supporting therapeutic interventions with enhanced efficacy and reduced side effects.
- Biological Research and Analysis: In research settings, microscale engineering contributes to studying cellular behavior, organ-on-a-chip models, and understanding fundamental biological processes at unprecedented resolutions. These platforms mimic physiological conditions more accurately than traditional methods, advancing our understanding of disease mechanisms and drug responses.
Mastering the key concepts and applications of microscale engineering in bioengineering equips researchers and engineers with the tools to innovate and address complex challenges in healthcare and biological research. As technology continues to evolve, the integration of engineering principles with biological insights will drive the development of transformative solutions that improve health outcomes and deepen our understanding of living systems.
Analytical Skills and Critical Thinking in Microscale Engineering
Analytical skills and critical thinking are not just desirable but essential competencies for mastering microscale engineering in bioengineering. This interdisciplinary field demands the ability to dissect complex data, critically evaluate experimental outcomes, and make informed decisions that drive the design, optimization, and application of microscale devices in biomedical contexts.
Developing Analytical Skills:
- Data Interpretation and Experimental Design: Effective data interpretation involves more than just understanding numerical results; it requires extracting meaningful insights that can guide the development of microscale devices. Engineers must be adept at applying statistical methods, employing data visualization techniques, and adhering to robust experimental design principles. These skills enable them to draw reliable conclusions, identify trends, and make data-driven decisions that optimize device performance.
- Problem-Solving in Device Design: Critical thinking plays a crucial role in overcoming challenges encountered during the design and optimization of microscale devices. Engineers must analyze a multitude of factors including material properties (such as biocompatibility and mechanical stability), fluid dynamics within microchannels, and the interface between biological systems and engineered materials. This analytical approach allows for the identification of design flaws, the troubleshooting of technical issues, and the proposal of innovative solutions that enhance device functionality and reliability.
- Evaluation of Device Performance: Assessing the performance of microscale devices requires a comprehensive approach to measurement and validation. Engineers employ analytical tools and develop rigorous validation protocols to assess parameters such as sensitivity, specificity, and reproducibility. By comparing device performance against established benchmarks and industry standards, engineers ensure consistency in results and validate the effectiveness of their designs in real-world applications.
Enhancing Critical Thinking:
- Innovative Design and Iterative Improvement: Critical thinking drives iterative design processes where engineers continuously refine prototypes based on feedback from experimental data, user requirements, and stakeholder input. This iterative approach fosters innovation by systematically addressing performance limitations, improving device functionalities, and adapting designs to meet evolving biomedical needs. It encourages engineers to explore novel solutions, push technological boundaries, and optimize designs for enhanced performance and reliability.
- Intgration of Multidisciplinary Knowledge: Microscale engineering in bioengineering often necessitates collaboration across diverse fields such as biology, chemistry, materials science, and biomedical engineering. Critical thinking enables engineers to integrate insights from these disciplines, leverage interdisciplinary approaches, and develop holistic solutions to complex biological and engineering challenges. This integrative mindset not only enriches the design process but also fosters a deeper understanding of the biological context in which microscale devices operate, leading to more effective and impactful innovations.
- Ethical and Societal Considerations: Beyond technical prowess, critical thinking in microscale engineering encompasses ethical considerations and societal impacts. Engineers must navigate ethical dilemmas related to patient safety, regulatory compliance, and the equitable distribution of healthcare technologies. By critically evaluating these factors, engineers ensure responsible innovation, advocate for ethical practices, and contribute to the development of healthcare solutions that are accessible, safe, and beneficial to society as a whole.
Analytical skills and critical thinking are foundational to mastering microscale engineering in bioengineering. These competencies empower engineers and researchers to navigate the complexities of device development, enhance experimental rigor, and drive transformative advancements in healthcare delivery. As technological advancements continue to evolve, the cultivation of analytical and critical thinking abilities will remain indispensable for addressing global health challenges and pioneering innovations that improve human health and well-being through bioengineering solutions. By honing these skills, engineers not only advance the frontiers of biomedical technology but also contribute to a future where precision medicine and personalized healthcare are increasingly achievable goals.
Practical Applications and Case Studies
Practical applications and case studies illustrate the real-world impact of microscale engineering in bioengineering, showcasing how innovative technologies are transforming healthcare diagnostics, therapeutic interventions, and biological research. These examples highlight the versatility and efficacy of microscale devices in addressing complex biomedical challenges.
Key Practical Applications:
- Point-of-Care Diagnostics: Microscale engineering enables the development of portable diagnostic devices that deliver rapid and accurate results at the point of care. For example, lab-on-a-chip technologies integrate sample preparation, analysis, and detection on a single microfabricated platform, facilitating early disease detection and treatment monitoring without the need for centralized laboratory facilities.
- Therapeutic Devices and Drug Delivery Systems: Bioengineered microdevices play a pivotal role in targeted drug delivery systems and therapeutic interventions. Microscale technologies allow for precise control over drug release kinetics, enabling localized delivery to specific tissues or cells while minimizing systemic side effects. Examples include microfluidic devices for controlled drug release and implantable microelectrodes for neural stimulation.
- Organ-on-a-Chip Models: Organ-on-a-chip models replicate physiological conditions of human organs within microscale platforms, offering a more accurate representation of tissue function and disease mechanisms compared to traditional cell culture models. These models facilitate drug testing, toxicity screening, and disease modeling, accelerating drug discovery and personalized medicine approaches.
Case Studies:
- Microfluidic COVID-19 Testing Devices: During the COVID-19 pandemic, microfluidic technologies played a crucial role in developing rapid antigen tests and molecular diagnostics for SARS-CoV-2 detection. These microscale devices enabled high-throughput testing with minimal sample volumes, contributing to global efforts in pandemic control and public health surveillance.
- Neural Interfaces for Prosthetic Control: Advanced neural interfaces, including microelectrode arrays and neural implants, enable bidirectional communication between prosthetic devices and the nervous system. These microscale devices translate neural signals into actionable commands for prosthetic limbs or restore sensory feedback, improving quality of life for individuals with limb loss or neurological disorders.
- 3D Bioprinting and Tissue Engineering: 3D bioprinting technologies utilize microscale deposition techniques to fabricate complex tissue constructs with precise spatial control over cell placement and scaffold materials. These bioengineered tissues can be used for regenerative medicine applications, including tissue repair, organ transplantation, and personalized implants tailored to patient-specific anatomical needs.
Practical applications and case studies demonstrate the transformative potential of microscale engineering in bioengineering, driving innovation across healthcare, biomedical research, and therapeutic development. By leveraging microscale technologies, engineers and researchers continue to pioneer new solutions that address global health challenges, improve patient outcomes, and advance our understanding of human biology. As microscale engineering evolves, ongoing research and development efforts will further expand the capabilities and applications of these innovative technologies in shaping the future of healthcare delivery and biomedical sciences.
Conclusion:
In conclusion, the practical applications and case studies of microscale engineering in bioengineering underscore its profound impact on healthcare and biomedical research. By harnessing the capabilities of microfabrication techniques and advanced materials, engineers have developed innovative solutions that enhance diagnostics, improve therapeutic outcomes, and deepen our understanding of biological systems. From portable diagnostic devices for point-of-care testing to complex organ-on-a-chip models and precision drug delivery systems, microscale technologies continue to revolutionize how we approach healthcare challenges.
The versatility and efficacy of microscale devices are evident in their ability to replicate physiological conditions, facilitate personalized medicine approaches, and accelerate drug discovery processes. Case studies such as microfluidic COVID-19 testing devices during the pandemic and neural interfaces for prosthetic control exemplify the transformative potential of microscale engineering in addressing global health crises and enhancing quality of life for patients.
Looking ahead, ongoing advancements in microscale engineering will further drive innovation in healthcare delivery, biomedical research, and therapeutic interventions. As researchers explore new applications and refine existing technologies, the integration of microscale engineering with biological sciences promises to shape the future of medicine and contribute to sustainable healthcare solutions worldwide. By fostering interdisciplinary collaboration and nurturing critical thinking skills, the field of microscale engineering in bioengineering continues to pave the way for groundbreaking discoveries and improvements in human health.
In this dynamic landscape, mastering the principles and applications of microscale engineering equips engineers and researchers with the tools to tackle complex challenges, drive innovation, and make meaningful contributions to the advancement of biomedical technology. As we navigate towards a future where precision medicine and personalized healthcare are increasingly achievable goals, microscale engineering stands at the forefront of transformative change, poised to redefine the boundaries of what is possible in healthcare and biological sciences.