Applying the Principles of Heat Transfer in Mechanical Engineering Assignments
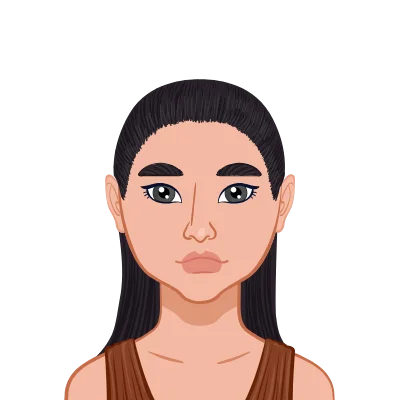
Heat transfer plays a pivotal role in the field of mechanical engineering, serving as a fundamental and indispensable aspect that underpins the functionality of a myriad of systems and devices. At its core, heat transfer involves the movement of thermal energy from one object or substance to another due to temperature differences. This phenomenon is central to the efficient operation and design of countless mechanical systems, impacting diverse industries and applications.
In the realm of mechanical engineering, a comprehensive understanding of heat transfer is crucial for optimizing the performance of machinery and ensuring the longevity of components. Completing your Heat Transfer assignment will further solidify your grasp of these fundamental principles. One of the key areas where heat transfer is of paramount importance is in the design and operation of heat exchangers. These devices are ubiquitous in industries such as power generation, chemical processing, and HVAC systems. Heat exchangers facilitate the efficient transfer of thermal energy between fluids, enabling processes like cooling, heating, and heat recovery. Without an adept grasp of heat transfer principles, engineers would struggle to design effective and energy-efficient heat exchangers, compromising the overall efficiency of these systems.
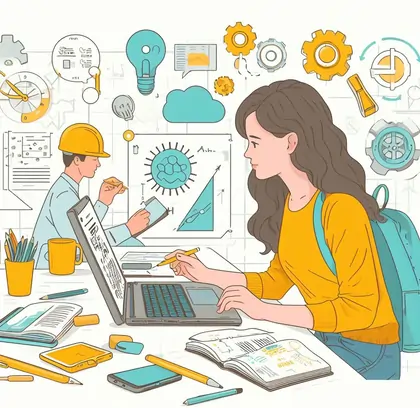
Moreover, heat transfer is instrumental in the development and advancement of thermal systems. Whether it be the design of engines for automobiles, aircraft propulsion systems, or industrial furnaces, a nuanced understanding of heat transfer mechanisms is imperative. In the automotive industry, for instance, optimizing the heat transfer within an engine is critical for enhancing fuel efficiency and minimizing environmental impact. Engineers must consider factors such as conduction through engine components, convection in the cooling system, and radiation from the exhaust system. The intricate interplay of these heat transfer mechanisms influences the overall performance and durability of the engine.
The relevance of heat transfer extends beyond the traditional domains of mechanical engineering, finding application in cutting-edge technologies and emerging fields. In the realm of electronics, heat transfer is a critical consideration for the design and functionality of electronic devices. As electronic components become more compact and powerful, managing heat dissipation becomes a significant challenge. Thermal management solutions, informed by principles of heat transfer, are essential to prevent overheating and ensure the reliability of electronic systems. The semiconductor industry, in particular, relies on sophisticated heat transfer techniques to enhance the efficiency and lifespan of integrated circuits.
Furthermore, the aerospace industry heavily depends on heat transfer principles for the design of aircraft and spacecraft. The extreme conditions encountered during space exploration or high-speed flight necessitate meticulous consideration of heat transfer mechanisms. Engineers must address challenges related to thermal protection, aerodynamic heating, and temperature differentials in the vacuum of space. The ability to effectively manage heat transfer ensures the safety and success of missions, from the re-entry of spacecraft into Earth's atmosphere to the operation of propulsion systems.
In the realm of renewable energy, heat transfer principles are instrumental in the design and optimization of solar thermal systems. These systems harness solar energy to generate heat, which can then be converted into electricity or used for heating applications. A profound understanding of how heat is transferred from sunlight to a working fluid, and subsequently to a power cycle or a heating system, is essential for maximizing the efficiency of solar technologies. Without a solid foundation in heat transfer, engineers would struggle to develop sustainable and economically viable solutions for harnessing solar energy.
In conclusion, the significance of heat transfer in mechanical engineering cannot be overstated. It is the linchpin that connects theoretical knowledge to real-world applications, influencing the design, efficiency, and reliability of mechanical systems across diverse industries. From the intricate components of an internal combustion engine to the outer reaches of space exploration, heat transfer is a universal principle that shapes the trajectory of innovation and progress in mechanical engineering. As technology continues to advance, the mastery of heat transfer principles will remain at the forefront of engineering endeavors, ensuring the continued evolution of mechanical systems and their seamless integration into the fabric of modern society.
Understanding Heat Transfer in Mechanical Engineering
In the realm of mechanical engineering, a profound understanding of heat transfer is essential for designing and optimizing systems that involve the exchange of thermal energy. Heat transfer encompasses three fundamental mechanisms: conduction, convection, and radiation, each playing a distinctive role in various mechanical applications.
Conduction, the first mechanism, involves the transfer of heat through a material without any apparent movement of the material itself. This process is governed by the molecular vibrations within a substance. In mechanical systems, conduction is evident in scenarios such as the transfer of heat through solid components like engine blocks or structural elements. For instance, in an internal combustion engine, conduction is critical for ensuring uniform temperature distribution across components, promoting efficient combustion and minimizing wear. Engineers must consider the thermal conductivity of materials to design components that facilitate or resist heat transfer as needed.
Convection, the second mechanism, occurs when heat is transferred through the movement of fluids—liquids or gases. This can take place through natural convection, where fluid motion is induced by temperature differences, or forced convection, where external means like pumps or fans drive fluid movement. Convection is prominent in applications such as cooling systems for electronic devices, HVAC systems, and liquid-cooled engines. In an automotive radiator, for instance, hot coolant flows through the radiator tubes, and heat is transferred to the surrounding air through forced convection, ensuring efficient cooling of the engine.
The third mechanism, radiation, involves the transfer of heat through electromagnetic waves and does not require a medium for propagation. While conduction and convection are primarily associated with solids and fluids, radiation is crucial in scenarios where direct physical contact is not possible. In mechanical engineering, radiation is prevalent in systems such as solar panels, where sunlight is absorbed and converted into thermal energy. Additionally, radiation plays a role in the design of spacecraft, where heat is dissipated into space in the absence of a medium for conduction or convection.
Practical applications of heat transfer principles are abundant in mechanical engineering, with one of the most notable being heat exchangers. These devices facilitate the efficient exchange of thermal energy between fluids at different temperatures, finding applications in diverse industries, including power generation, chemical processing, and refrigeration. The design of a heat exchanger requires careful consideration of factors such as fluid flow rates, temperatures, and the choice of materials to optimize heat transfer efficiency.
In thermal systems design, another crucial area, engineers apply heat transfer principles to create systems that efficiently generate, transfer, and utilize thermal energy. This encompasses the design of engines, furnaces, and heating, ventilation, and air conditioning (HVAC) systems. In the aerospace industry, understanding heat transfer is vital for designing thermal protection systems that shield spacecraft during re-entry into Earth's atmosphere, where extreme temperatures are encountered.
In conclusion, a nuanced comprehension of heat transfer mechanisms is foundational to the practice of mechanical engineering. Whether it be the efficient cooling of electronic devices, the optimization of energy conversion in engines, or the design of advanced aerospace systems, heat transfer principles are omnipresent. Engineers leverage these principles to overcome challenges, enhance efficiency, and ensure the reliability and performance of mechanical systems across diverse applications. As technological advancements continue, the mastery of heat transfer in mechanical engineering remains an ongoing pursuit, driving innovation and shaping the future of the discipline.
Practical Applications in Mechanical Engineering Assignments
Practical applications of heat transfer principles within the context of mechanical engineering assignments span a wide array of scenarios, each contributing to the optimization and efficiency of various systems. One prominent application lies in the design and analysis of heat exchangers. These devices serve as linchpins in industries such as power generation, chemical processing, and refrigeration, facilitating the controlled transfer of thermal energy between fluids at different temperatures. Mechanical engineering assignments related to heat exchangers often involve intricate calculations and considerations, demanding a thorough understanding of conduction, convection, and radiation. Students may be tasked with optimizing heat exchanger designs to enhance efficiency, exploring different materials to maximize thermal conductivity, or addressing challenges related to fluid flow rates and pressure drops.
Thermal systems design represents another key practical application area in mechanical engineering assignments. Engineers working on assignments in this domain delve into the development of engines, furnaces, and HVAC systems. For example, assignments may require students to design an energy-efficient internal combustion engine by applying heat transfer principles to optimize combustion processes and manage thermal stresses. In HVAC systems, students might be tasked with developing solutions to enhance heat transfer in heat exchangers or improving the overall energy efficiency of the system. Such assignments not only demand theoretical knowledge but also necessitate creative problem-solving skills to address real-world challenges in thermal systems.
Energy efficiency in mechanical systems emerges as a critical theme in assignments related to heat transfer principles. Students may explore ways to enhance energy efficiency in diverse applications, from automotive engines to industrial processes. For instance, an assignment might involve analyzing the heat transfer mechanisms within an automotive engine to minimize energy losses and improve fuel efficiency. Alternatively, students might be challenged to propose innovative solutions for reducing heat losses in industrial processes, contributing to sustainability goals. These assignments push students to think critically about the practical implications of heat transfer principles, emphasizing the role of mechanical engineers in creating energy-efficient solutions for a sustainable future.
Beyond traditional mechanical engineering applications, assignments may also venture into emerging fields, such as the application of heat transfer principles in electronic devices. With the continuous miniaturization of electronic components, managing heat dissipation has become a paramount concern. Assignments in this domain may require students to design effective thermal management solutions for electronic devices, considering factors like heat conduction through circuit boards, convection in cooling systems, and the impact of radiation on temperature control. These practical applications highlight the interdisciplinary nature of heat transfer principles, showcasing their relevance in addressing challenges at the intersection of mechanical engineering and electronics.
In conclusion, practical applications of heat transfer principles in mechanical engineering assignments are diverse and far-reaching, reflecting the integral role of these principles in optimizing systems across various industries. As students engage with assignments related to heat exchangers, thermal systems design, and energy efficiency, they not only deepen their theoretical understanding but also cultivate the problem-solving skills necessary for real-world engineering challenges. The practical application of heat transfer principles underscores their significance in shaping the efficiency, sustainability, and innovation of mechanical systems in contemporary engineering practice.
Challenges and Problem-Solving
Navigating the intricacies of heat transfer assignments presents students with a host of challenges that require both theoretical understanding and practical problem-solving skills. One common challenge revolves around the complex nature of mathematical modeling in heat transfer scenarios. Students often grapple with the intricacies of differential equations and mathematical formulations that describe heat conduction, convection, and radiation. The application of these equations to real-world problems, such as predicting temperature distributions in a heat exchanger or analyzing transient heat conduction in a material, demands a high level of mathematical proficiency, posing a hurdle for many learners.
Another significant challenge arises from the diverse materials and boundary conditions encountered in heat transfer problems. Assignments may require students to analyze heat transfer in materials with varying thermal conductivities or explore scenarios with complex geometries and boundary conditions. The ability to adapt theoretical knowledge to diverse contexts and employ appropriate mathematical methods becomes paramount. This challenge not only tests students' comprehension of heat transfer principles but also their capacity to apply these principles in nuanced and context-specific ways.
In practical applications like heat exchanger design, students often encounter challenges related to fluid dynamics. Understanding the behavior of fluids, including heat transfer coefficients, pressure drops, and flow patterns, is integral to optimizing heat exchanger performance. Students must grapple with the complexities of fluid flow, especially in scenarios involving multiphase flows or turbulent conditions. These challenges demand a comprehensive understanding of fluid mechanics alongside heat transfer principles, adding a layer of complexity to assignments.
Furthermore, assignments focusing on transient heat transfer phenomena present students with challenges related to time-dependent processes. Analyzing temperature changes over time in response to varying conditions requires a deep understanding of transient heat conduction and convection. Students must navigate the intricacies of time-dependent boundary conditions and the transient response of materials, showcasing their ability to apply heat transfer principles dynamically.
Thermal radiation, as a distinct mode of heat transfer, introduces another layer of complexity. Assignments involving radiation heat transfer often pose challenges due to their non-intuitive nature and the need to consider factors like emissivity and absorption coefficients. Students must grapple with the mathematical expressions governing radiative heat transfer and apply them in scenarios such as designing systems that leverage solar radiation for energy conversion.
Collaborative aspects of heat transfer assignments also present challenges for students, particularly when working on team projects or tackling interdisciplinary problems. Integrating knowledge from related fields, such as fluid mechanics, materials science, and thermodynamics, can be demanding. Effective communication and collaboration are essential, yet students may face difficulties in synthesizing information from various disciplines to address the multifaceted aspects of heat transfer problems.
In conclusion, the challenges in heat transfer assignments underscore the interdisciplinary and multifaceted nature of this field within mechanical engineering. From mathematical complexities and diverse material considerations to fluid dynamics and transient phenomena, students must navigate a spectrum of challenges that go beyond theoretical understanding. These challenges not only contribute to the educational rigor of heat transfer assignments but also mirror the intricacies inherent in real-world engineering problems, preparing students for the complexities they will encounter in their future careers.
Conclusion
In conclusion, the exploration of Applying the Principles of Heat Transfer in Mechanical Engineering Assignments reveals the intricate and indispensable role that heat transfer plays in the realm of mechanical engineering. The journey through conduction, convection, and radiation underscores the foundational principles that govern the exchange of thermal energy and shape the efficiency of diverse systems.
Our journey through conduction, convection, and radiation underscored the essential mechanisms governing the exchange of thermal energy. Practical applications revealed the critical role of heat exchangers, thermal system design, and the pursuit of energy efficiency in various industries. Heat exchanger assignments challenge students to optimize fluid dynamics and material properties, emphasizing the real-world complexities of heat exchange scenarios.
In thermal system design, students bridge theory and application, grappling with combustion processes, fluid flow, and temperature management. These assignments cultivate the ability to design systems that balance efficiency and reliability—a hallmark of proficient mechanical engineers. The focus on energy efficiency throughout assignments highlights the transformative potential of applying heat transfer principles to address global sustainability goals.
However, mastering heat transfer comes with challenges. The complexities of mathematical modeling, diverse materials, fluid dynamics intricacies, and dynamic transient phenomena form hurdles for students. Collaborative aspects add complexity, reflecting the interdisciplinary nature of real-world engineering challenges.
In essence, applying heat transfer principles in mechanical engineering assignments extends beyond textbook knowledge. It demands a synthesis of theory, mathematical acumen, and practical problem-solving skills. As students grapple with these challenges, they are not just solving assignments; they are preparing for the multifaceted demands of the engineering profession.