Thermodynamics in Renewable Energy: Analysis and Design Assignments!
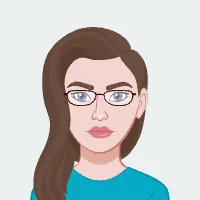
In the ever-evolving landscape of mechanical engineering, the convergence of thermodynamics and renewable energy emerges as a captivating and dynamic realm. As we venture deeper into the foundational principles of thermodynamics, the applications within the domain of renewable energy reveal themselves as both pivotal and intricate. This blog post endeavors to navigate this fascinating intersection, shedding light on the symbiotic relationship between thermodynamics and renewable energy. The focus will be on unraveling the complexities inherent in this connection, with a keen emphasis on the analytical and design facets that assume a pivotal role in shaping the future of sustainable engineering. Whether you're seeking help with thermodynamics assignments or aiming to explore the intricate nexus between thermodynamics and renewable energy, this blog post serves as a guiding beacon in your academic and professional journey.
The principles of thermodynamics serve as the bedrock upon which the edifice of mechanical engineering stands. In the pursuit of harnessing renewable energy, understanding the core tenets of thermodynamics becomes not just a prerequisite but a guiding light. The intricate dance between energy, heat transfer, and the laws of thermodynamics shapes the landscape within which engineers strive to innovate and propel the world towards a greener future. It is within this complex tapestry that we find the nexus between thermodynamics and renewable energy – a nexus that demands exploration and comprehension.
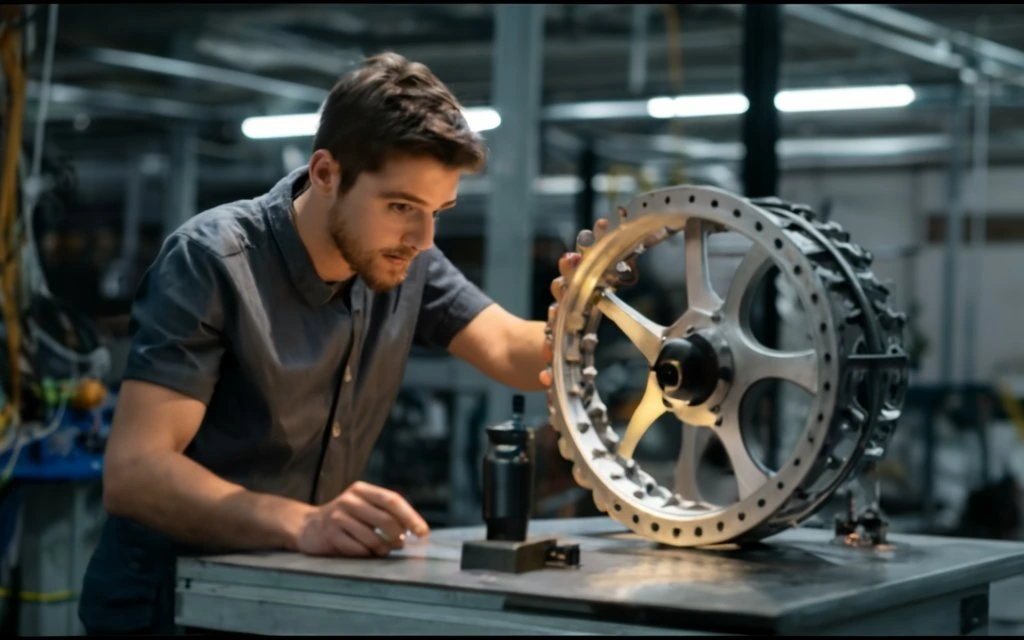
Analyzing this symbiotic relationship reveals that thermodynamics is not merely a theoretical construct confined to textbooks but a dynamic force steering the advancements in renewable energy technology. From solar and wind to geothermal sources, thermodynamic principles underpin the efficiency and functionality of these systems. The very essence of energy conversion, storage, and utilization in renewable technologies is governed by the laws that define thermodynamics. The task at hand, therefore, is to dissect and comprehend these applications, unraveling the secrets that make renewable energy systems both challenging and transformative.
At the heart of this exploration lie the analysis and design aspects that form the backbone of innovative engineering solutions. By delving into these facets, we unearth the practical implications of thermodynamics in renewable energy. Analysis assignments beckon mechanical engineering students to apply theoretical knowledge to real-world scenarios – evaluating the efficiency of a solar power plant, optimizing heat transfer in geothermal systems, and scrutinizing the dynamics of wind turbines. These assignments serve not only as academic exercises but as stepping stones for students to grapple with the challenges posed by the rapidly evolving field of renewable energy.
Moving beyond analysis, the realm of design introduces its own set of challenges. From conceptualizing more efficient wind turbines to devising thermodynamically optimized solar collectors, students are tasked with not only understanding the intricacies of thermodynamics but applying this understanding to create tangible solutions. These design challenges serve as crucibles for creativity and critical thinking, instilling in students the ability to innovate in the pursuit of sustainable energy solutions.
In conclusion, the interplay between thermodynamics and renewable energy represents a captivating journey in mechanical engineering. This exploration is not just an academic pursuit but a critical endeavor that shapes the trajectory of our planet's energy future. By unraveling the complexities, embracing the challenges, and fostering a deep understanding of the relationship between thermodynamics and renewable energy, we equip the engineers of tomorrow to lead the charge towards a cleaner, more sustainable world.
Understanding the Basics:
Before delving into the intricate world of analysis and design assignments, it is imperative to embark on a journey revisiting the fundamental principles that underpin the entire realm of thermodynamics. This moment of reflection serves as a crucial foundation, ensuring a comprehensive understanding of the core concepts before applying them to the challenges of renewable energy. At the heart of this revisitation lies the exploration of key notions such as energy transfer, heat exchange, and the immutable laws of thermodynamics.
Energy transfer, a fundamental concept in thermodynamics, is the crux of understanding how energy flows and transforms within a system. Whether in the form of mechanical work, heat, or other manifestations, comprehending the principles governing energy transfer is paramount to unraveling the complexities of renewable energy systems. The nuances of how energy can be harnessed, converted, or dissipated form the basis for innovative solutions that propel the field forward.
Heat exchange, another pivotal concept, plays a crucial role in the efficiency and functionality of numerous engineering systems. From the optimization of heat transfer in solar collectors to the intricate mechanisms within geothermal systems, a nuanced grasp of heat exchange principles is indispensable. This aspect of thermodynamics becomes the linchpin for engineers seeking to harness and manipulate heat energy in sustainable ways, paving the way for advancements in renewable energy technologies.
The laws of thermodynamics, often considered the governing commandments of the physical world, provide the framework within which engineers navigate the intricacies of energy systems. The first law, emphasizing the conservation of energy, lays the groundwork for understanding energy transformations. The second law, delving into the inevitability of entropy, guides engineers in optimizing processes for efficiency. These laws form the compass by which design and analysis assignments are undertaken, ensuring that the solutions crafted adhere to the fundamental principles that govern the behavior of energy.
As we revisit these fundamental concepts, we set the stage for a comprehensive exploration of their applications in renewable energy. This preparatory step is not a mere formality but a deliberate strategy to equip engineers with the knowledge and insight necessary to tackle the challenges that lie ahead. Armed with a renewed understanding of energy transfer, heat exchange, and the laws of thermodynamics, engineers are poised to embark on a transformative journey where theory meets application, and innovation becomes the driving force in the pursuit of sustainable energy solutions.
The Role of Thermodynamics in Renewable Energy:
The pivotal role of thermodynamics in the realm of renewable energy sources, such as solar, wind, and geothermal, cannot be overstated. At the core of these sustainable energy systems lie principles deeply rooted in thermodynamics, serving as the guiding force that determines their efficiency and overall performance. To comprehend the intricate dance between thermodynamics and renewable energy, it is imperative to explore how these fundamental principles govern every facet of these innovative technologies.
Solar energy, one of the most widely embraced forms of renewable power, exemplifies the direct application of thermodynamics. Photovoltaic cells, central to solar panels, convert sunlight into electricity through a process dictated by thermodynamic principles. Understanding the efficiency of these cells, the impact of temperature differentials, and the optimization of energy conversion processes requires a nuanced appreciation of thermodynamic concepts. Engineers and researchers delve into the intricacies of these systems, leveraging thermodynamics to enhance the effectiveness of solar energy capture and utilization.
Wind energy, harnessed through wind turbines, is another domain where thermodynamics plays a defining role. The conversion of kinetic energy from moving air into electrical power follows the principles of thermodynamics. Engineers meticulously analyze factors such as blade design, wind speed, and turbine efficiency, applying thermodynamic principles to maximize energy extraction from the wind. Through the lens of thermodynamics, the efficiency of wind energy systems is optimized, making strides towards a more sustainable and eco-friendly energy landscape.
Geothermal energy, derived from the Earth's internal heat, operates within the principles of thermodynamics as well. By tapping into the natural heat reservoirs beneath the Earth's surface, geothermal power plants convert thermal energy into electricity. The efficiency of these systems is intricately tied to thermodynamic considerations, influencing the design and operational parameters of geothermal facilities. Thermodynamics guides engineers in mitigating energy losses, improving heat transfer processes, and ultimately enhancing the overall performance of geothermal energy systems.
To illustrate the real-world impact of thermodynamics in renewable energy, case studies and examples become invaluable tools. Analyzing instances where thermodynamic principles have been successfully applied in harnessing clean energy provides a tangible perspective. Whether it's the design of a more efficient solar collector, the optimization of a wind farm layout, or the enhancement of geothermal power plant performance, these case studies offer insights into the practical application of thermodynamics in solving complex challenges within the renewable energy landscape.
In conclusion, the synergy between thermodynamics and renewable energy is a symbiotic relationship that drives innovation and sustainability. Through a profound understanding of thermodynamic principles, engineers and researchers propel the efficiency and performance of solar, wind, and geothermal systems, paving the way for a cleaner and more sustainable energy future. The exploration of case studies and real-world examples further underscores the transformative potential of thermodynamics in shaping the landscape of clean and eco-friendly energy solutions.
Analysis Assignments:
Immersing mechanical engineering students in specific analysis assignments that bridge theoretical knowledge with real-world applications is a cornerstone in fostering a profound understanding of thermodynamics within the context of renewable energy systems. These assignments serve as dynamic platforms, challenging students to apply thermodynamic principles in practical scenarios, thus preparing them for the complexities of the evolving engineering landscape.
One notable analysis assignment involves the assessment of the efficiency of a solar power plant. Students are tasked with unraveling the intricacies of energy conversion from sunlight to electricity, contemplating factors such as the efficiency of photovoltaic cells, the impact of varying solar radiation, and the overall system losses. By applying thermodynamic principles, students gain hands-on experience in evaluating the performance of solar power plants, considering both theoretical aspects and the practical constraints inherent in harnessing solar energy.
Optimizing heat transfer in geothermal systems presents another compelling analysis assignment. Geothermal energy, drawn from the Earth's internal heat, relies on effective heat transfer mechanisms for optimal performance. Students engage in the nuanced evaluation of heat exchange processes within geothermal power plants, considering factors such as fluid properties, geological variations, and the dynamics of heat extraction. This assignment not only deepens their understanding of thermodynamics but also equips them with the skills to enhance the efficiency of geothermal energy systems, addressing challenges faced in real-world applications.
These analysis assignments go beyond the confines of traditional classroom exercises, immersing students in the practical intricacies of renewable energy technologies. The hands-on nature of these assignments instills problem-solving skills and encourages critical thinking as students grapple with the complexities of optimizing energy systems. Furthermore, the application of thermodynamic principles in these assignments fosters a holistic understanding of the interconnectedness between theory and practice.
In the broader context, these assignments empower students to contribute meaningfully to the ongoing global transition towards sustainable energy. By addressing real-world challenges faced by solar power plants and geothermal systems, students not only enhance their academic proficiency but also cultivate a sense of responsibility in tackling the environmental issues that define our era. As they navigate these analysis assignments, mechanical engineering students are not just solving problems on paper; they are actively engaging in the transformative work of shaping a cleaner, more sustainable future through the application of thermodynamic principles to renewable energy systems.
Design Challenges:
Transitioning from analysis to design challenges marks a pivotal phase in the education of mechanical engineering students, offering them a platform to showcase creativity and critical thinking within the dynamic arena of renewable energy technology. These design challenges extend beyond theoretical exploration, tasking students with the conceptualization of solutions that push the boundaries of efficiency and sustainability. One such challenge involves the design of a more efficient wind turbine—a task that necessitates a profound understanding of aerodynamics, structural engineering, and, significantly, thermodynamics. Students grapple with optimizing blade designs, considering wind flow dynamics, and maximizing energy extraction while adhering to real-world constraints. This not only sharpens their technical skills but also instills a deep appreciation for the multidisciplinary nature of renewable energy engineering.
Similarly, designing a thermodynamically optimized solar collector constitutes another intriguing challenge. Here, students delve into the intricacies of heat transfer, materials science, and system integration to craft a solar collector that maximizes energy absorption and conversion. They explore innovations in design parameters such as surface coatings, reflectors, and thermal storage systems, all while keeping thermodynamic principles at the forefront. This assignment propels students beyond the theoretical realm, encouraging them to think holistically about the real-world application of their designs and the potential impact on overall system efficiency.
These design challenges serve as catalysts for innovation, inspiring students to think beyond conventional solutions and push the boundaries of what is achievable in renewable energy technology. The integration of thermodynamics into the design process ensures that the solutions generated are not only innovative but also grounded in the fundamental principles that govern energy systems. By marrying theoretical knowledge with creative problem-solving, students gain a comprehensive understanding of the intricate balance between efficiency, sustainability, and practical application in the field of renewable energy.
Moreover, these design challenges foster a mindset of continuous improvement and adaptation—an essential quality in an ever-evolving technological landscape. Students learn to navigate the complexities of real-world engineering problems, understanding that the pursuit of more efficient wind turbines and thermodynamically optimized solar collectors contributes to the ongoing global effort to transition towards sustainable energy solutions. As they engage with these challenges, mechanical engineering students are not merely designing for the present; they are crafting solutions that have the potential to shape the future of renewable energy technology, reflecting the transformative power of creative thinking informed by thermodynamic principles.
Conclusion:
As our exploration of the intricate relationship between thermodynamics and renewable energy draws to a close, it is imperative to underscore the profound significance of thermodynamics in shaping the trajectory of the future of sustainable energy. Thermodynamics, beyond being a theoretical framework, emerges as the guiding force that propels innovation and progress in the realm of renewable energy. It is the compass that navigates engineers and researchers through the complexities of harnessing clean and efficient energy from sources such as solar, wind, and geothermal.
The assignments undertaken by mechanical engineering students in the domain of thermodynamics are not mere academic exercises; they are transformative endeavors that hold the potential to contribute significantly to a more sustainable and environmentally conscious world. The analysis assignments, where students dissect the efficiency of solar power plants or optimize heat transfer in geothermal systems, are not isolated endeavors confined to the classroom. They represent a critical step in the journey towards developing solutions that address real-world challenges in renewable energy technology.
Moving beyond analysis to design challenges, students are not only crafting more efficient wind turbines and thermodynamically optimized solar collectors; they are actively participating in the creation of technologies that can redefine the energy landscape. By instilling a sense of responsibility and purpose, these assignments encourage students to see themselves as agents of change—contributors to a future where the reliance on fossil fuels diminishes, and sustainable energy sources flourish.
In encouraging students to view these assignments as contributions, we emphasize the interconnectedness between their academic pursuits and the broader global imperative for sustainability. Each analysis and design task undertaken becomes a tangible step towards a future where energy systems are not only efficient but also ecologically responsible. Thermodynamics serves as the bridge between the theoretical and the practical, guiding students to develop solutions that transcend the classroom and have a lasting impact on the environment.
As we wrap up this exploration, the call to action is clear: embrace thermodynamics as a catalyst for change. Recognize the pivotal role that these assignments play in steering our world towards a more sustainable future. The knowledge gained and innovations crafted by mechanical engineering students today have the potential to shape the energy landscape of tomorrow. By imparting this perspective, we empower the engineers of the future to approach their work not merely as a career but as a vocation—one that holds the promise of a cleaner, greener, and more harmonious coexistence with our planet.