Workforce and Sustainability Assignment Challenges in CIM Systems
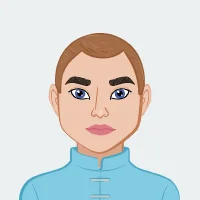
In the rapidly evolving landscape of manufacturing, Computer Integrated Manufacturing (CIM) has emerged as a cornerstone of modern industrial practices, driving significant improvements in production efficiency, flexibility, and overall competitiveness. CIM represents a sophisticated framework that leverages advanced technologies to streamline and optimize manufacturing processes through the seamless integration of various systems and components. This blog aims to explore the multifaceted nature of CIM, providing an in-depth look at its core components, recent technological advancements, and practical applications within contemporary manufacturing environments.
CIM encompasses a range of technologies and methodologies designed to enhance the precision and efficiency of manufacturing operations. By integrating components such as Computer-Aided Design (CAD), Computer-Aided Manufacturing (CAM), and Computer Numerical Control (CNC) machines, CIM facilitates a cohesive and automated production process. Additionally, the incorporation of robotics, material handling systems, and quality control mechanisms further contributes to the seamless operation of manufacturing systems.
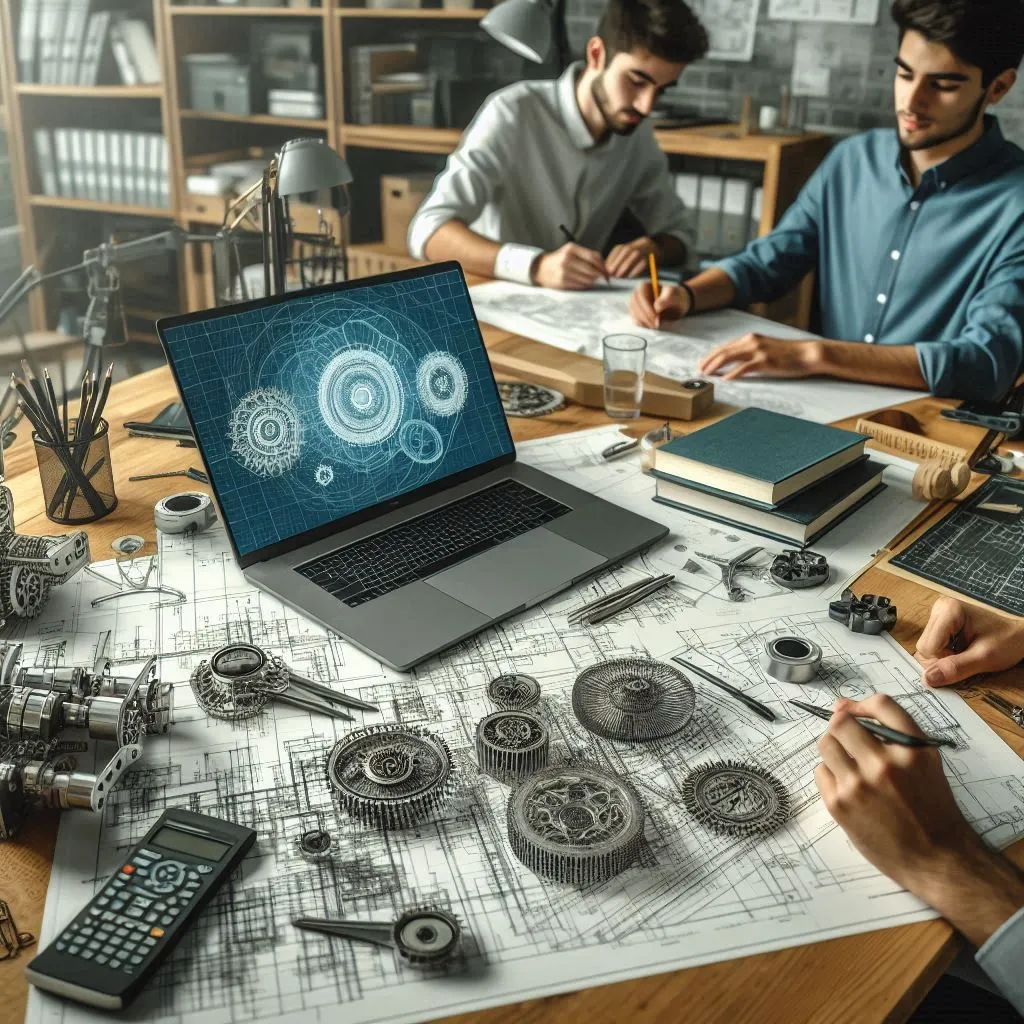
The technological landscape of CIM is continually advancing, with emerging trends such as Industry 4.0, the Internet of Things (IoT), Artificial Intelligence (AI), Big Data, and Cyber-Physical Systems playing a crucial role in enhancing its capabilities. Understanding these advancements allows students to grasp how CIM systems are evolving to meet the demands of modern manufacturing, including increased automation, real-time data analysis, and improved system responsiveness.
Practical integration of CIM within manufacturing environments involves not only the design and implementation of complex systems but also the analysis and optimization of their performance. By utilizing simulation software to model and assess the efficacy of CIM systems, students can identify opportunities for cost reduction, resource optimization, and performance enhancement.
Moreover, the impact of CIM extends beyond technical considerations to encompass broader issues such as workforce dynamics and sustainability. Analyzing how CIM affects job roles, skill requirements, and environmental practices provides a holistic view of its implications for the manufacturing sector.
By delving into these aspects, students can approach assignments related to CIM with a comprehensive understanding of its principles and applications. This knowledge not only prepares them for academic challenges but also equips them with the practical skills necessary to navigate and excel in the evolving field of manufacturing technology. For those seeking manufacturing processes assignment help, this foundational understanding is crucial in tackling complex problems and optimizing manufacturing solutions.
Understanding CIM System Design and Integration
A well-designed Computer Integrated Manufacturing (CIM) system is crucial for enhancing production efficiency, flexibility, and overall effectiveness in modern manufacturing environments. It integrates a range of technological components to create a streamlined and automated manufacturing process. Here’s an expanded overview of the key elements involved in designing an effective CIM system and how to approach its integration. For students seeking mechanical engineering assignment help, understanding these elements is essential for tackling assignments related to CIM systems and applying theoretical knowledge to practical scenarios.
Key Elements of CIM System Design
- Computer-Aided Design (CAD): CAD systems are fundamental in the CIM framework, enabling detailed and precise design and visualization of products. They allow engineers and designers to create accurate digital models of products, which serve as the basis for further manufacturing processes. The integration of CAD into a CIM system ensures that design specifications are accurately translated into production instructions, reducing errors and improving the overall quality of the end product.
- Computer-Aided Manufacturing (CAM): CAM systems take the designs created in CAD and convert them into machine-readable instructions. These instructions guide CNC machines and other automated equipment in the production process. CAM systems enhance the efficiency of manufacturing by automating complex machining processes, improving precision, and reducing production time. The seamless integration of CAM with CAD ensures that design changes are swiftly and accurately reflected in the manufacturing process.
- Computer Numerical Control (CNC) Machines: CNC machines play a critical role in automating the machining processes. They execute the instructions generated by CAM systems with high precision and repeatability. CNC machines are used for various manufacturing tasks, including cutting, drilling, and milling. Their integration into a CIM system allows for greater control over the manufacturing process, leading to improved consistency and reduced manual intervention.
- Robotics: Robotics are integral to the automation of tasks such as assembly, material handling, and packaging. They enhance the flexibility of manufacturing operations by performing repetitive tasks with high speed and accuracy. In a CIM system, robotics are programmed to interact seamlessly with other components, such as CNC machines and material handling systems, to optimize the production workflow and reduce manual labor.
- Material Handling Systems: These systems are responsible for managing the movement, storage, and retrieval of materials within the manufacturing environment. Effective material handling systems ensure that materials are delivered to the right place at the right time, minimizing delays and disruptions in the production process. Integrating material handling systems with other CIM components helps streamline operations and improve overall efficiency.
- Quality Control Mechanisms: Quality control mechanisms are essential for ensuring that the final products meet the specified standards and specifications. These mechanisms can include automated inspection systems, sensors, and feedback loops that monitor and control the quality of products throughout the manufacturing process. Integrating quality control with CIM systems helps maintain high standards of product quality and reduces the likelihood of defects.
Designing and Integrating CIM Systems
To design an effective CIM system, students and professionals should follow a structured approach:
- Create a Flowchart or Block Diagram: Developing a flowchart or block diagram is an essential step in visualizing the integration and data flow between different CIM components. This diagram should illustrate how each component interacts and the flow of information and materials throughout the system. It helps in understanding the overall system architecture and identifying potential areas for improvement.
- Discuss the Role of Each Component: Each component in a CIM system has a specific role in the manufacturing process. For instance, CAD systems are responsible for design, CAM systems translate designs into instructions, and CNC machines execute those instructions. Understanding the role of each component and how they communicate with each other is crucial for designing an effective CIM system.
- Identify Essential Software and Hardware: Selecting the right software and hardware is critical for the successful implementation of a CIM system. Software requirements may include CAD and CAM applications, control software for CNC machines, and robotics programming tools. Hardware requirements include CNC machines, robotics, sensors, and material handling equipment.
- Analyze Potential Bottlenecks: Analyzing potential bottlenecks involves identifying areas where the CIM system may experience inefficiencies or delays. Common bottlenecks may include limitations in processing speed, inadequate communication between components, or constraints in material handling. Identifying these issues early on allows for the development of solutions to mitigate their impact.
- Propose Solutions to Enhance System Efficiency: Based on the analysis of potential bottlenecks, propose solutions to improve the efficiency of the CIM system. Solutions may include upgrading hardware, optimizing software algorithms, enhancing communication protocols, or redesigning workflow processes. Implementing these solutions helps ensure that the CIM system operates at its full potential and meets the desired performance standards.
By understanding and effectively implementing these elements and strategies, students can design, analyze, and optimize CIM systems that are well-suited to the demands of modern manufacturing environments. This comprehensive approach not only enhances academic performance but also prepares students for practical challenges in the field of manufacturing technology.
Integrating CIM with Industry 4.0 Technologies
Industry 4.0 signifies a revolutionary shift in manufacturing, characterized by the integration of cutting-edge technologies that transform traditional manufacturing processes into highly automated, data-driven systems. This new era of manufacturing emphasizes interconnectedness, real-time data exchange, and intelligent decision-making. Integrating Industry 4.0 technologies with Computer Integrated Manufacturing (CIM) systems enhances their capabilities, leading to more efficient, responsive, and adaptive manufacturing environments. Here’s an in-depth look at how these technologies augment CIM systems and the challenges and strategies associated with their integration:
Key Industry 4.0 Technologies Enhancing CIM Systems
- Internet of Things (IoT): The Internet of Things (IoT) connects various devices, sensors, and systems within a manufacturing environment, enabling real-time data exchange and control. IoT facilitates seamless communication between different components of a CIM system, such as CAD, CAM, CNC machines, and robotics. This connectivity allows for continuous monitoring and adjustment of manufacturing processes, leading to improved efficiency and reduced downtime. For example, IoT-enabled sensors can provide real-time feedback on machine performance, allowing for predictive maintenance and minimizing unexpected breakdowns.
- Artificial Intelligence (AI): Artificial Intelligence (AI) enhances CIM systems by improving decision-making processes and enabling predictive maintenance. AI algorithms can analyze large volumes of data generated by CIM systems to identify patterns and anomalies that may not be immediately apparent. This capability allows for proactive adjustments and optimizations in manufacturing processes. For instance, AI-driven analytics can forecast equipment failures before they occur, enabling timely maintenance and reducing production interruptions.
- Big Data: Big Data involves the collection and analysis of vast amounts of data generated by manufacturing systems. By leveraging Big Data, CIM systems can gain valuable insights into production performance, process efficiency, and quality control. Analyzing data from various sources, such as machine sensors, production logs, and quality reports, enables manufacturers to identify trends, optimize processes, and make data-driven decisions. For example, analyzing data on machine performance can help identify the root causes of inefficiencies and guide improvements in the manufacturing process.
- Cyber-Physical Systems (CPS): Cyber-Physical Systems (CPS) integrate physical processes with digital control systems, creating a cohesive and responsive manufacturing environment. CPS combines physical components, such as machines and sensors, with digital systems, such as software and control algorithms, to monitor and control manufacturing processes in real time. This integration enhances the adaptability and flexibility of CIM systems, allowing for dynamic adjustments based on real-time data. For instance, CPS can enable automated adjustments to production schedules based on real-time demand fluctuations.
Examples of Successful Integration
- Siemens: Siemens has successfully integrated Industry 4.0 technologies into its CIM systems, leveraging IoT and Big Data to enhance manufacturing processes. Siemens' Digital Factory division uses IoT sensors to monitor and control machinery in real time, enabling predictive maintenance and process optimization. Additionally, Siemens utilizes Big Data analytics to gain insights into production performance and drive continuous improvement.
- General Electric (GE): General Electric (GE) has implemented Industry 4.0 technologies in its manufacturing operations through its "Brilliant Factory" initiative. GE employs AI and IoT to enhance machine performance and reduce downtime. By using AI-driven analytics and IoT sensors, GE can predict equipment failures, optimize production schedules, and improve overall operational efficiency.
- Bosch: Bosch has integrated Cyber-Physical Systems (CPS) into its CIM systems to create highly responsive manufacturing environments. Bosch's use of CPS allows for real-time monitoring and control of production processes, leading to increased flexibility and adaptability. Bosch's CPS-enabled systems can automatically adjust production parameters based on real-time data, improving efficiency and reducing waste.
Challenges and Strategies for Integration
- Challenge: Data Security and Privacy Integrating Industry 4.0 technologies introduces concerns about data security and privacy, as sensitive information is transmitted and stored across interconnected systems. To address this challenge, manufacturers should implement robust cybersecurity measures, including encryption, access controls, and regular security audits, to protect data from unauthorized access and breaches.
- Challenge: System Compatibility Integrating New Industry 4.0 technologies with existing CIM systems may face compatibility issues, particularly if legacy systems are involved. To overcome this challenge, manufacturers should invest in interoperable technologies and platforms that support seamless integration. Additionally, gradual implementation and phased upgrades can help ensure compatibility and reduce disruption.
- Challenge: Skills and Training The adoption of advanced technologies requires a skilled workforce capable of managing and utilizing these systems effectively. Manufacturers should invest in training and development programs to equip employees with the necessary skills and knowledge to work with Industry 4.0 technologies. Collaboration with technology providers and educational institutions can also help bridge the skills gap.
- Challenge: High Initial Costs The initial investment in Industry 4.0 technologies can be substantial, posing a challenge for manufacturers with limited budgets. To address this, manufacturers should evaluate the long-term benefits and return on investment (ROI) of these technologies. Implementing pilot projects and scaling up gradually can also help manage costs and demonstrate the value of the investment.
By understanding and effectively integrating Industry 4.0 technologies with CIM systems, manufacturers can achieve significant improvements in automation, data analysis, and system responsiveness. These advancements not only enhance the efficiency and flexibility of manufacturing processes but also position companies to compete successfully in the dynamic landscape of modern manufacturing.
Simulation and Optimization of CIM Systems
Simulation and optimization are essential aspects of Computer Integrated Manufacturing (CIM) systems, enabling manufacturers to model, analyze, and enhance their manufacturing processes before physical implementation. These techniques provide valuable insights into how CIM systems perform under various conditions and help identify opportunities for improvement. Here’s an in-depth look at the simulation and optimization of CIM systems, including their significance, methodologies, and practical applications:
Significance of Simulation and Optimization
Simulation involves creating a virtual model of a CIM system to replicate real-world manufacturing processes. By running simulations, manufacturers can observe how the system behaves under different scenarios, identify potential issues, and test solutions without disrupting actual production. This approach allows for a thorough evaluation of the system’s performance, efficiency, and reliability before making physical changes.
Optimization focuses on enhancing the performance of a CIM system by adjusting parameters to achieve specific objectives, such as minimizing costs, reducing production time, or improving resource utilization. Optimization techniques help in fine-tuning the system to meet desired performance metrics and operational goals.
Methodologies for Simulation and Optimization
1. Creating Simulation Models: To simulate a CIM system, manufacturers must develop a detailed model that represents all critical components and processes. This model includes:
- Process Flow: Mapping out the sequence of operations, from material input to finished product output.
- Component Interactions: Illustrating how different components, such as CAD systems, CAM systems, CNC machines, and robotics, interact and communicate.
- Resource Management: Incorporating elements like material handling, storage, and inventory management into the model.
Simulation software, such as Siemens Tecnomatix or AnyLogic, can be used to build and run these models. The software allows users to simulate various production scenarios, analyze the flow of materials and information, and evaluate the system’s performance.
2. Simulation Scenarios: Running simulations involves testing the CIM system under different conditions, including:
- Normal Operations: Evaluating system performance under typical production conditions.
- Peak Loads: Analyzing how the system handles high demand or increased production rates.
- Failure Conditions: Testing how the system responds to equipment failures, bottlenecks, or other disruptions.
These scenarios help identify potential weaknesses, assess the impact of various factors, and develop strategies for mitigating risks.
3. Optimization Techniques: Optimization involves adjusting system parameters to improve performance. Key techniques include:
- Parameter Tuning: Adjusting settings such as machine speeds, production schedules, and inventory levels to achieve optimal performance.
- Resource Allocation: Allocating resources efficiently, including labor, materials, and equipment, to maximize productivity and reduce waste.
- Cost Analysis: Evaluating the cost implications of different scenarios and making adjustments to minimize expenses while maintaining quality and efficiency.
Optimization algorithms, such as linear programming, genetic algorithms, or simulated annealing, can be used to find the best configuration for the CIM system.
4. Sensitivity Analysis: Sensitivity analysis involves studying how changes in one part of the CIM system affect overall performance. By examining the impact of variations in parameters, such as machine uptime or material flow rates, manufacturers can understand the system’s responsiveness and identify critical factors that influence performance.
Sensitivity analysis helps in pinpointing areas where small adjustments can lead to significant improvements and informs decision-making for system enhancements.
Practical Applications of Simulation and Optimization
- Improving Production Efficiency: Simulation and optimization can help identify bottlenecks and inefficiencies in the manufacturing process. By analyzing different configurations and operational strategies, manufacturers can optimize production schedules, reduce downtime, and enhance overall efficiency.
- Reducing Costs: Through optimization, manufacturers can minimize production costs by optimizing resource allocation, reducing waste, and improving process efficiency. Simulation allows for evaluating cost implications before implementing changes, helping in making cost-effective decisions.
- Enhancing Quality Control: Simulation can be used to test different quality control strategies and assess their impact on product quality. By optimizing quality control mechanisms, manufacturers can ensure that products meet specified standards and reduce the likelihood of defects.
- Designing Flexible Manufacturing Systems: Simulation enables the design of flexible manufacturing systems that can adapt to changing production requirements. By modeling different scenarios, manufacturers can develop systems that are responsive to market demands and capable of handling varying production volumes.
- Implementing New Technologies: When integrating new technologies into a CIM system, simulation helps in evaluating their impact and ensuring compatibility with existing components. This approach allows for a smooth transition and minimizes the risks associated with adopting new technologies.
Simulation and optimization are powerful tools for enhancing CIM systems, offering valuable insights into system performance and opportunities for improvement. By creating detailed simulation models, running various scenarios, and applying optimization techniques, manufacturers can achieve higher efficiency, reduce costs, and improve overall production quality. These methodologies not only support better decision-making but also enable the development of more adaptable and responsive manufacturing environments.
Analyzing the Impact of CIM on Workforce and Sustainability
The integration of Computer Integrated Manufacturing (CIM) systems profoundly influences both the workforce and sustainability practices within manufacturing environments. By automating processes and enhancing operational efficiency, CIM systems bring significant changes to job roles, skill requirements, and environmental impact. This analysis explores these effects and provides strategies to address potential challenges, ensuring that the implementation of CIM systems benefits both the workforce and sustainability goals.
Impact on the Workforce
1. Job Roles and Skill Requirements:
- Shift in Job Roles: The adoption of CIM systems often leads to a shift in job roles within manufacturing organizations. Traditional manual tasks are automated, resulting in a decreased need for manual labor while increasing the demand for roles focused on system management, maintenance, and optimization. Workers may transition from hands-on manufacturing tasks to positions involving system monitoring, data analysis, and process improvement.
- Skills Development: As CIM systems integrate advanced technologies, there is a growing need for employees with specialized skills. Workers need to be proficient in operating and troubleshooting automated systems, understanding complex software, and analyzing data. This shift necessitates investment in training programs to equip employees with the necessary skills to work effectively with CIM technologies.
2. Job Displacement and Creation:
- Displacement: Automation and advanced technologies can lead to job displacement, particularly for roles that involve repetitive or routine tasks. Employees in these roles may face job insecurity as their tasks are automated.
- Creation of New Roles: Conversely, CIM systems create new job opportunities in areas such as system design, programming, data analysis, and maintenance. These roles require higher skill levels and offer opportunities for career advancement. Companies should focus on reskilling and upskilling programs to help displaced workers transition into new roles.
3. Workforce Dynamics:
- Increased Collaboration: CIM systems often promote increased collaboration between different departments, such as engineering, production, and IT. Workers need to communicate effectively and work together to ensure the successful integration and operation of CIM systems.
- Enhanced Job Satisfaction: Automation of mundane tasks can lead to increased job satisfaction as employees focus on more engaging and intellectually stimulating activities. However, successful implementation requires clear communication and support to manage the transition effectively.
Impact on Sustainability
1. Environmental Impact:
- Energy Consumption: CIM systems can lead to significant reductions in energy consumption by optimizing production processes and improving the efficiency of machinery. Advanced control systems can minimize energy waste and reduce the overall environmental footprint of manufacturing operations.
- Waste Reduction: Automation and precise control of manufacturing processes help in minimizing material waste. CIM systems enable more accurate production planning, reduce defects, and improve material utilization, leading to less waste generation.
2. Resource Utilization:
- Efficient Resource Management: CIM systems enhance resource management by optimizing material handling, inventory management, and production scheduling. Improved resource utilization reduces the need for excess raw materials and minimizes the impact on natural resources.
- Sustainable Practices: Implementing CIM systems encourages the adoption of sustainable practices, such as recycling and reuse of materials. Advanced manufacturing technologies can facilitate the development of products with lower environmental impacts and promote circular economy principles.
3. Product Life Cycle:
- Extended Product Life: CIM systems contribute to the production of high-quality products with longer lifespans. Enhanced quality control and precision in manufacturing processes reduce the likelihood of defects and extend the life cycle of products, contributing to sustainability.
- End-of-Life Management: Advanced CIM systems can support effective end-of-life management by facilitating the recycling and disposal of products. Manufacturers can design products with disassembly and recyclability in mind, reducing environmental impact at the end of the product’s life.
Strategies for Addressing Challenges
1. Workforce Training and Development:
- Training Programs: Invest in comprehensive training programs to help employees acquire the skills needed to operate and manage CIM systems effectively. Focus on both technical skills and soft skills, such as problem-solving and collaboration.
- Career Transition Support: Provide career transition support for employees affected by job displacement. Offer reskilling opportunities and career counseling to help workers move into new roles within the organization or the broader industry.
2. Communication and Change Management:
- Clear Communication: Ensure clear and transparent communication about the changes brought by CIM systems. Involve employees in the transition process and address their concerns to build trust and facilitate acceptance.
- Change Management: Implement change management strategies to support employees through the transition. Provide resources, support, and incentives to encourage adoption and adaptation to new roles and technologies.
3. Sustainability Initiatives:
- Environmental Policies: Develop and implement environmental policies that promote energy efficiency, waste reduction, and sustainable resource management. Regularly review and update these policies to reflect advancements in CIM technology and sustainability goals.
- Continuous Improvement: Foster a culture of continuous improvement in sustainability practices. Encourage innovation and collaboration to identify new ways to enhance environmental performance and reduce the ecological footprint of manufacturing operations.
The integration of CIM systems has significant implications for both the workforce and sustainability practices within manufacturing environments. By understanding and addressing the impacts on job roles, skill requirements, and environmental factors, manufacturers can effectively manage the transition to CIM systems. Through strategic planning, investment in training, and commitment to sustainability, organizations can leverage CIM technologies to enhance operational efficiency, create new opportunities, and contribute to a more sustainable future.
Conclusion
The integration of Computer Integrated Manufacturing (CIM) systems marks a transformative shift in the manufacturing landscape, offering profound benefits in terms of operational efficiency, resource management, and environmental sustainability. By embracing CIM, manufacturers can achieve enhanced precision, automation, and flexibility, leading to streamlined processes and optimized production.
For the Workforce: CIM systems necessitate a shift in job roles and skill requirements, creating both challenges and opportunities. While automation may lead to job displacement in certain areas, it also generates new roles that demand advanced technical skills. To navigate these changes successfully, companies must invest in comprehensive training and reskilling programs, fostering a workforce adept at managing and utilizing CIM technologies. Effective communication and change management strategies are crucial in ensuring a smooth transition and maintaining workforce morale.
For Sustainability: CIM systems contribute significantly to sustainability by reducing energy consumption, minimizing waste, and improving resource utilization. The ability to precisely control manufacturing processes and optimize resource management supports environmental goals and promotes sustainable practices. By focusing on energy efficiency, waste reduction, and end-of-life management, manufacturers can enhance their environmental performance and support broader sustainability initiatives.
In summary, CIM systems represent a pivotal advancement in manufacturing technology, offering substantial benefits for both the workforce and sustainability. By addressing the impacts on job roles and environmental practices with thoughtful strategies and ongoing support, manufacturers can harness the full potential of CIM systems. Embracing these technologies not only drives operational excellence but also supports a more sustainable and resilient manufacturing future.